Quick Look
Grade Level: 11 (10-12)
Time Required: 1 hours 15 minutes
Lesson Dependency: None
Subject Areas: Chemistry, Physics
NGSS Performance Expectations:
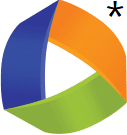
HS-PS1-3 |
Summary
Polymers are a vital part of our everyday lives and nearly all consumer products have a plastic component of some variation. Students explore the basic characteristics of polymers through the introduction of two polymer categories: thermoplastics and thermosets. During teacher demos, students observe the unique behaviors of thermoplastics. The fundamentals of thermoset polymers are discussed, preparing them to conduct the associated activity in which they create their own thermoset materials and mechanically test them. At the conclusion of this lesson-activity pair, students understand the basics of thermoplastics and thermosets, which may entice their interest in polymer engineering.Engineering Connection
Polymer production (thermosets and thermoplastics) is a multibillion dollar industry in which chemists and polymer engineers collaborate to develop products. For successful polymer production, a deep understanding of polymer physics and the unique behavior of polymers is required so that the material properties are fully exploited. Polymer chemists come up with chemical pathways to create polymers. Polymer engineers understand how these new polymers behave so they can maximize mechanical properties for particular applications. And, the process engineers who control large-scale polymer production are aware of how polymers behave differently than their simpler counterparts so that polymer reactions and subsequent production of particular polymer parts can be mass produced. Collectively, these specialists produce products that ultimately improve our everyday lives.
Learning Objectives
After this lesson, students should be able to:
- Define a polymer and list everyday examples (at least one thermoset and one thermoplastic).
- Describe three unique polymer behaviors.
- In their own words, describe enthalpic and entropic interactions.
- Explain shear thinning and shear thickening, focusing on the overlying idea not on the details, and how shear thinning pertains to polymers.
- Describe, in their own words, thermoplastics and thermosets.
- Explain, using the classical analogy of a bridge, how to make a stiff and flexible thermoset.
Educational Standards
Each TeachEngineering lesson or activity is correlated to one or more K-12 science,
technology, engineering or math (STEM) educational standards.
All 100,000+ K-12 STEM standards covered in TeachEngineering are collected, maintained and packaged by the Achievement Standards Network (ASN),
a project of D2L (www.achievementstandards.org).
In the ASN, standards are hierarchically structured: first by source; e.g., by state; within source by type; e.g., science or mathematics;
within type by subtype, then by grade, etc.
Each TeachEngineering lesson or activity is correlated to one or more K-12 science, technology, engineering or math (STEM) educational standards.
All 100,000+ K-12 STEM standards covered in TeachEngineering are collected, maintained and packaged by the Achievement Standards Network (ASN), a project of D2L (www.achievementstandards.org).
In the ASN, standards are hierarchically structured: first by source; e.g., by state; within source by type; e.g., science or mathematics; within type by subtype, then by grade, etc.
NGSS: Next Generation Science Standards - Science
NGSS Performance Expectation | ||
---|---|---|
HS-PS1-3. Plan and conduct an investigation to gather evidence to compare the structure of substances at the bulk scale to infer the strength of electrical forces between particles. (Grades 9 - 12) Do you agree with this alignment? |
||
Click to view other curriculum aligned to this Performance Expectation | ||
This lesson focuses on the following Three Dimensional Learning aspects of NGSS: | ||
Science & Engineering Practices | Disciplinary Core Ideas | Crosscutting Concepts |
Plan and conduct an investigation individually and collaboratively to produce data to serve as the basis for evidence, and in the design: decide on types, how much, and accuracy of data needed to produce reliable measurements and consider limitations on the precision of the data (e.g., number of trials, cost, risk, time), and refine the design accordingly. Alignment agreement: | The structure and interactions of matter at the bulk scale are determined by electrical forces within and between atoms. Alignment agreement: | Different patterns may be observed at each of the scales at which a system is studied and can provide evidence for causality in explanations of phenomena. Alignment agreement: |
International Technology and Engineering Educators Association - Technology
-
Assess a technology that minimizes resource use and resulting waste to achieve a goal.
(Grades
9 -
12)
More Details
Do you agree with this alignment?
-
Critue whether existing and proposed technologies use resources sustainably.
(Grades
9 -
12)
More Details
Do you agree with this alignment?
-
Evaluate how technologies alter human health and capabilities.
(Grades
9 -
12)
More Details
Do you agree with this alignment?
State Standards
Texas - Science
-
describe how the macroscopic properties of a thermodynamic system such as temperature, specific heat, and pressure are related to the molecular level of matter, including kinetic or potential energy of atoms;
(Grades
9 -
12)
More Details
Do you agree with this alignment?
-
analyze and explain everyday examples that illustrate the laws of thermodynamics, including the law of conservation of energy and the law of entropy.
(Grades
9 -
12)
More Details
Do you agree with this alignment?
Worksheets and Attachments
Visit [www.teachengineering.org/lessons/view/uoh_polymer_lesson01] to print or download.Pre-Req Knowledge
Students should have some chemistry background. This lesson could be taught as part of a high school chemistry course, or in another class while students are concurrently taking a high school chemistry course. It is also relevant for students who have previously completed a high school chemistry course.
Introduction/Motivation
With technology all around us, sometimes we fail to realize how one particular type of material has completely shaped our lives. The seat that you sit on, the inside panels that make up your car, the shampoo that you wash your hair with, and even the oil that helps your engine run—each contains a special material: polymers. But what are polymers? They are often overlooked and seldom taught in any depth so it is understandable that we may have to dig deeper when it comes to polymers. A better understanding of polymers helps us engineer better products for the future.
Today we are going to learn about the basics of polymers and then go into a bit of detail about two classes of polymers: thermoplastics and thermosets. This learning includes a demo that shows the unique behavior that thermoplastic polymers can exhibit and another that shows what makes a thermoset. As we go through each demo, I will emphasize why it is important and make engineering connections to our everyday world.
As we go through this lesson, remember that polymers are long chains. Knowing this will help you understand why they behave the way they do in our class demonstrations. Our goal is to understand why polymers behave the way they do, so pay close attention to our discussion. Then, follow-up with the Bridging to Polymers: Thermoset Lab activity to have students further their learning by mechanically testing their own thermosets.
Lesson Background and Concepts for Teachers
Note: The teacher demonstrations require some advance preparation; see instructions below for details. The two sets of demonstrations show each of the Weissenberg, Barus, Kaye and defying gravity effects. One demo uses a polyvinyl alcohol (PVA) solution and requires a power drill and a glass rod. The other demo requires rubber bands and a large wooden rod.
If time does not permit the demo preparations, show students these online videos instead: https://www.youtube.com/watch?v=nX6GxoiCneY and https://www.youtube.com/watch?v=w0Ib25BPR2I).
Before starting the lesson, it is helpful for the teacher to read through the Close Encounters of the Polymer Kind Presentation prompts and notes on each slide of the PowerPoint® file and through the Close Encounters Worksheet to review essential background information to supplement that which is provided below. If time does not permit going through the entire lesson background, read only the slides and present students with the Close Encounters of the Polymer Kind Lesson Handout for Students, which provides a full summary of the background information contained in the lesson. Then use either the worksheet or handout as a graded assignment.
Materials List for Demos
- polyvinyl alcohol (PVA), $34 for 25 grams https://www.sigmaaldrich.com/catalog/product/aldrich/363146?lang=en®ion=US
- borax, $75 for 100 grams https://www.sigmaaldrich.com/catalog/product/sigma/ht1002?lang=en®ion=US
- glass rod
- thermometer
- mass scale
- hot plate
- 200 ml beaker
- 100 ml beaker
- power drill
- liquid food coloring
- wooden dowel
- 25+ thin rubber bands
- computer with internet connection and projection capabilities
Making the PVA Solution
- Heat 100 ml of water to 80 °C and add 4 grams PVA..
- Stir the solution over heat while mixing until the PVA is dissolved. It is a slow process.
- In another beaker, add 20 ml water and 1 gram borax.
- Mix the two solutions and allow time to cross-link (overnight if possible).
- Add a color dye if desired, to make the demo more colorful.
Discussion with Students
Note: The presentation includes embedded videos on slides 7, 12, 15 and 21. In order to play the videos on each slide, you must download the videos: Three Viscoelastic Effects and Defying Gravity, as well as the PowerPoint® file, and save them in the same folder on your computer. Alternatively, view the videos via YouTube at the URLs provided in the Additional Multimedia Support section.
Set up the presentation to display in the classroom. Hand out the Close Encounters Worksheet. Go through the slides, guided by the script provided below and text in the "notes" section of each slide. Embedded assessment questions are provided in italics below; allow discussion time before supplying the answers.
Slide 1 - Today we are going to begin a polymer unit, titled "Close Encounters of the Polymer Kind." The title refers to a popular sci-fi movie about UFOs and is appropriate because—for most of you—this topic is a foreign concept.
Slide 2 - Starting with the basics, we will discuss what a polymer is and introduce two categories of polymers, thermoplastics and thermosets. Next, we will discuss the cool behaviors of thermoplastics and the fundamentals that go into making a good thermoset. Then, we will perform a thermoset activity and, using our knowledge from the lesson, make our own thermosets.
Slide 3 - So what is a polymer? (Answer: A polymer is a long chain of repeating chemical units.)
Slide 4 - If we look deeper, we see that polymers are typically separated into two types: thermosets, such as tires and car bumpers, and thermoplastics, such as water bottles, which are often made of polyethylene, as mentioned earlier.
Can anyone tell me the difference between a thermoplastic and thermoset? (Pause to wait for both terms to be defined if possible; emphasize the "set" portion of the word "thermoset.") Here's a tip: If something is "set," can it be changed? (If students are unable to answer, move forward in the lesson. The answers are on the next two slides.)
Slide 5 - A thermoset is a polymer in which the final shape of the product becomes set, or cured, due to an irreversible chemical reaction. What is meant by curing? (Answer: To become tougher or harden.) Curing is a term for the chemical reaction that takes place to form the final 3D network that sets the shape.
What is a covalent bond? (Answer: A chemical bond that shares electrons.)
Slide 6 - Thermoplastic is a linear polymer whose final shape can be changed through heating the material and melting it so that other shapes can be formed.
What are some typical thermoplastics materials you encounter? (Answer: Water and soda bottles.)
To understand polymers, physical entanglements are an important concept to know. Imagine a bowl of spaghetti with all the noodles entangled due to their long lengths. This analogy helps us to imagine the physical entanglements at the molecular level.
Slide 7 - Now that we have introduced two categories of polymers, let's talk about some of the cool behaviors of thermoplastics!
Demo 1: The Weissenberg Effect
(In front of the class, have a beaker of water, a beaker of prepared PVA solution and a power drill with a glass rod as the bit.)
(While showing students the glass-bit drill and the beaker of water, ask them to make predictions.) What do you think will happen when we spin the drill in the beaker of water? Will the water move towards or away from the rod? (Expect answers to vary.)
(After students answer, conduct the demonstration by accelerating the glass drill bit in the beaker of water, demonstrating that the water moves away from the rod.) What do you think will happen when we place the rod in the PVA solution? Will the solution move towards or away from the rod? (Conduct the experiment using the PVA solution, showing that the PVA solution moves up the rod.)
What did you observe in the Weissenberg demo? (Answer: Polymer goes towards and up the rod; water moves down and away.)
Thus, the Weissenberg Effect is the instance in which a polymer travels up a rod as it spins, in contrast to say water that moves away from the rod as it spins. Why does this happen? By looking more closely at the differences between long chain polymers and water, we can figure out why this is the case.
Polymer chains interact and entangle, similar to a pile of string. For the case of water, the water has a certain affinity to the rod. As the rod spins, the centrifugal effect throws the water molecule away from the rod. For polymers, however, many more interactions with the rod exist per molecule and so much more force is required to remove the polymer from the rod. The chains are also entangled, so as the rod tries to throw the chain off the rod, it has nowhere to go and begins to wrap around the rod. As the chain wraps around the rod, it drags more chains closer to the rod due to entanglements. This explains why polymers come close to the rod.
(Note: In front of the class, have ready a pile of rubber bands, mound of salt and a wooden dowel.)
Slide 8 - Now, let's look at a macroscopic example. In front of me are a pile of rubber bands and a pile of salt. Watch what happens when a wooden dowel is rotated in salt.
(Rotate the wooden dowel in the mound of salt; expect the salt to move away from the dowel.) What happened in this demo? (Answer: Salt, which represents water, moved away from the dowel.)
(Then rotate the wooden dowel in the mound of rubber bands; expect the rubber bands to wrap around the dowel.) What happened? (Answer: The rubber bands, which represent the polymer, wrap around the wooden dowel.)
This is an excellent example of what happens at the microscopic level. To put this in more scientific terms, let's learn some vocabulary so we can relate this to the polymer.
Entropy and Enthalpy
Polymers might be described as like people who are in close contact in a small room: they want plenty of room to stretch their arms and legs. Essentially, they want to increase entropy or create disorder of the current system.
If a person was standing in the room next to an enemy, s/he would be inclined to move; in this case, repulsion exists between the two people. This is analogous to enthalpy. Polymer chains also experience attraction and repulsion due to Van der Waals forces.
In the case of the polymer chain, their desire to increase their ability to stretch out is greater than their attraction and some net movement has to take place. Since they cannot move down the rod without running into other polymer chains, the only option is to move up.
Slide 9 - We have a balance between enthalpic interactions and entropic interactions. Enthalpic interactions are how much you like the person next to you or—in molecular terms—how much a molecule likes the molecule next to it. Entropic interactions are how close you are to the one sitting next to you. The closer you sit to each other, the less entropy (capacity for disorder) you have available and the more uncomfortable you are in terms of sitting on top of each other.
To understand the difference between enthalpic and entropic, let's use this classroom as an example. Everyone wants to have plenty of space so that they can relax, correct (like polymers do)? Yet, at the same time, you want to sit next to your friend, right? Well...
What if your friend gets too close? (Listen to student answers.) No matter how favorable the enthalpic interactions (that is, how much you like your friend), you still want to keep some minimum amount of space beween you.
What if the person getting close to you is your enemy? (Listen to student answers.) In essence, the distance at which you begin to feel uncomfortable is greater than if you are friends. You become repulsed by one another.
So how does this relate to polymers? (Listen to student answers.) Well, polymers are long chains that get entangled and wrapped around the dowel. As the dowel is rotated, the polymers (rubber bands) pull more and more toward the dowel due to entanglements (decreasing entropy). The polymers need to spread out, but to maximize the amount of space, the polymer can only go up.
Demo 2: Barus & Kaye Effects
The Barus Effect is similar to the Weissenberg Effect in that it can be explained by the balances of the entropic and enthalpic contributions. Think back to the YouTube video of the three effects. What happened in the Barus Effect? As the polymer exited the die (mold), it expanded and swelled. Figure 1 shows a general scheme of this effect.
If we consider the classroom analogy again, we can get a good idea of what is happening. You are in a classroom with many students and one of the walls moves in and out. As the wall slowly moves toward you, you are forced to move closer and closer to your classmates until you get become close enough that through either (or both) entropic or enthalpic contributions, you want more space and you begin to push back on the wall. Now imagine the door suddenly opens and you are allowed to leave. You are naturally going to pour out into the hallways and spread out. This is essentially what happens with the Barus effect.
Now imagine that you have a bunch of polymer chains in a confined space and a plunger slowly puts energy into the system forcing the polymer through a die. As it does this, the chains must move in closer together (decreasing entropy) and the chains align. The chains move through the die, while pushing on the walls. Once the chains exit the die, they are free to relax and stretch out again by swelling (increasing entropy).
Slide 10 - These next two effects are a bit more subtle, so let's watch a YouTube video demonstration (https://www.youtube.com/watch?v=nX6GxoiCneY). The first demo is of the Barus effect and causes what is known as "die swell." What is meant by "die"? (Listen to student answers.) When you have a thermoplastic, it can be melted and forced through a die (a mold) to give it a particular shape. For example, if I wanted to make polyethylene (PE) fibers, I could melt PE and force it through a small diameter die to get the fiber.
(With students, watch the Die Swell Portion (Barus Effect) of the video.)*
Why do you think the swelling occurs? (If students do not understand what is meant by swelling, rewind the video to show how the liquid stream is wider than the mouth of the bottle.) Notice how the liquid stream is wider than the mouth of the bottle; this is "swelling." (Give students time to think about this viscoelastic effect; do not provide the answer.)
Let's look at another classroom analogy to explain the Barus effect phenomenon. Let's say one of the walls can move in and out. It moves so far in that everyone is forced to touch each other. Do you get more or less comfortable as the wall moves in? (Let students discuss their answers for a couple of minutes.) Why? What happens to entropy? Is there more or less space? (Permit students to discuss their answers for a couple of minutes.) Unless you are all extremely good friends, you are probably a little uncomfortable at the point of the wall closing in on you. The entropy (the disorder) of the room has decreased, as less space available for the same number of people.
What if I open the door so you can leave? (Listen to student answers.) That's right, you would want to pour out, 'swell,' into the hallway and spread out very quickly.
Slide 11 - How does this classroom example relate to the video?
As an example, as the plunger (the classroom wall) is pushed in toward us, the molecules (the class) are forced into a confined space, which decreases entropy. As for the liquid, the molecules exit the die (in this case, the bottle) and increase entropy by spreading out, or swelling.
Which has more entropy: a polymer chain that is forced to be straight or a chain that is free to move? (Answer: A polymer that is forced to be straight has less entropy available to it, since it is confined.) In order to increase the entropy of the system, the polymer spreads out when exiting the die.
Explain to me why water does not swell after exiting the die. (Listen to student explanations.) Compare the state of entropy in the die and the one after the die. Is it different or roughly the same ? (Answer: It is the same since it is so small. A confined water molecule looks very similar to an unconfined molecule of water so it cannot tell the difference.)
Shear Thinning and Shear Thickening
Slide 12 - This next effect is a much debated topic so we are going to present just one theory as to why it happens. Take a moment to watch the video of the Kaye effect. Pay attention to how the material behaves when it hits the surface.
(Watch the Kaye Effect portion of the YouTube video; either review the Weissenberg Effert portion or skip to the Kaye Effect, which starts at 45 seconds.)
What happens during the Kaye Effect? (Give students time to discuss.) The stream of poured material "skips" off the surface of the mound. One of the theories as to why this happens is called shear thinning. To understand this concept, let's consider the "pool of cornstarch and water" experiment, commonly called "oobleck" (see https://www.youtube.com/watch?v=yHlAcASsf6U). The experiment demonstrates shear thickening—the exact opposite of what is happening in the Kaye Effect video. In the cornstarch example, the material gets stiffer as you run across it. In fact, the faster you run, the easier it is to cross the material. With shear thinning, the material gets softer as you run across it. The faster you run, the softer the material gets. Think of the floor; although it is solid, you would fall through it if you ran across it too fast if it was shear thinning.
Imagine that you are a long polymer chain. You have had sufficient time to relax and reach a state that minimizes your free energy (maximized entropy). This shape that you would be in would roughly resemble a sphere (think about why water droplets form). As you move about, you collide with other spheres roughly the same size as you, and it takes considerable time for you to go around each other. As stress (energy) is applied to the system, you are disturbed from your little sphere and are forced to stretch out as you collide with each other. You are not allowed time to relax back to the original state (time constraints), and you remain in this stretched state. You and your neighbors become aligned. You are no longer colliding with large spheres. Instead you are just sliding past each other. Your resistance to flow or viscosity is greatly decreased and you can appear to skip off the surface. Figure 2 illustrates this example.
Let's again refer to the classroom analogy. This time, we are running through the halls and a person in the back wants to move to the front. We consider two situations: one in which the person at the back pulls out and runs slowly to the front; and the other in which the person very quickly moves to the front.
In the first case, the student slowly goes through the group of students en route to the front. S/he is able to move around the other students and not disturb them.
In the second case, the student moves too quickly (that is, too sloppily) to skillfully move around the other students without colliding with them. If the rapidly moving student is too quick, the students being bumped into cannot recover from the collisions and return to their normal states of moving. They are disrupted, so to speak.
Slide 13 - Now extend this to polymers. We have established that polymers like to have their own space and curl up in a relaxed configuration. But when they begin to collide with each other more quickly than they can return back to their relaxed configuration, they get stretched out.
Once stretched, do you think it is easier or harder for polymers to slide past each other? (Give students time to discuss.) It is easier, which results in shear thinning.
Slide 14 - Viscosity is the resistance to flow. If it is difficult for the fluid to flow, it is said that the viscosity is high—or more viscous. A real-world example of this is paint, which is a type of thermoplastic polymer. When you paint something, you do not want the paint to be all runny and slide down the wall. However, you also do not want it to be too thick, making it difficult to apply. As you apply the brush to the wall, you apply force to the paint and it shear thins, making it easy to apply. When the polymer leaves the brush (and the applied force of the brush) it returns to its original state of being somewhat "thick" so as to not run down the wall.
Demo 3: Defying Gravity Effect
Slide 15 - What if I told you that I could defy gravity and remove material from the beaker without the syringe being in the beaker the whole time?
(Perform the demo described in the next paragraph, or watch the video at: https://www.youtube.com/watch?v=w0Ib25BPR2I.)
(Demo instructions: Have ready a syringe, a beaker of water and a beaker of PVA Solution. Place the syringe in the beaker of water and begin to slowly draw some out. As you slowly draw the water, ask students: Do you think I could continue to pull the water from the beaker while removing the syringe from the beaker? Then conduct the same experiment with the PVA. To do so, insert the tip of the syringe in the solution and begin to pull back on the syringe. While pulling back, remove the syringe tip from the PVA solution. You should be able to pull the PVA solution out while removing the syringe out of the beaker.)
What happened in the demo? Did I defy gravity? (Answer: Yes; the material could be removed from the beaker without the syringe being in it.) Why was this possible? (Note: Entanglements is the answer but hold off on revealing this just yet.)
Now, let's consider another macroscopic example. In front of me, I have a pile of rubber bands. I need to challenge a volunteer to try to pull one rubber band, and only one rubber band, from the middle of the pile. If you disturb any of the other rubber bands, you fail the challenge. Who would like to accept the challenge?
(Let one student try to pull one rubber band free from the pile.)
Could [name] do it? (Most probably not. If the student is able, mix up the rubber bands again and have him/her try again by picking a rubber band at random. Repeat until it is clear that if you pull one rubber band, you pull others with it. Essentially, as one rubber band is pulled, it drags others with it.)
Slide 16 - How does this relate? (Listen to student explanations.) A similar event happens in the syringe. As one polymer is pulled up, it drags others with it. When the syringe is initially in the solution, it "grabs" onto a bunch of other polymers. Once removed from the solution, those chains continue to drag other chains into the syringe.
Thermosets
Previously we discussed some of the molecular dynamics of thermoplastic systems including how the ability to relax affects a material's properties (think of shear thinning or shear thickening). Let's switch our focus to thermosets. Say we take the thermoplastic system we saw earlier through the Weissenberg Effect demo, but now we anchor the molecule in place every few points within the molecule. Now the molecules cannot move as well. Anchor a few more points, and the material gets stiffer as the molecules lose the ability to move around. A macroscopic (large scale) example of an anchored thermoset is a bridge, as shown in Figure 3. In the first illustration, the bridge has very few connections.
Do you think this bridge would be very stiff or somewhat flexible? (Answer: It is somewhat flexible.)
Now we take the exact same material, but make a few more connections.
What happens to the stiffness? (Answer: It goes up.)
If we shrink this to the molecular level, we have a thermoset system with different amounts of molecular "crosslinks," or connections. If we vary the amount of connections, we change the mechanical properties.
Now let's discuss how we can change the number of crosslinks at a molecular level. Figure 4 shows two difunctional molecules—that is, two sites within the molecule that are reactive towards each other. If they react, they can only create a linear chain of connections.
Is the polymer now linear if we have a thousand difunctional molecules (500 A and 500 B) and exchange a single "A" with a single trifunctional molecule (D)? (Give students time to discuss before moving on to the next questions.)
And, what happens if we exchange it for two trifunctional molecules? (Give students time to discuss before moving on to the next questions.)
What if we directly exchange 10 B molecules for 10 D molecules? (Give students time to discuss.)
What if we completely replace the 500 B molecules with 333 D molecules? (Give students time to discuss before explaining the exchange of molecules.)
Every A functional group can react with one D functional group—perfect number of connections due to a stoichiometric ratio.
What if we keep going and add 1,000 trifunctional molecules? Are enough difunctional molecules present to react with all of the trifunctional? (Answer: No, and now you have loose ends again, which causes the material to become flexible again.) This happens through a reaction between an epoxy (difunctional molecule) and an amine-bearing molecule that possesses more than two functional groups (shown in Figure 4). Common examples of epoxy resins are car bumpers or panels and wind turbine blades.
Slide 17 - Thermosets are polymer systems that have gone through a curing reaction and are "set" in their final shapes. A thermoset cannot be reshaped by heating. Thermosets are interesting because you can take a bunch of small reactive molecules, fill up a shape that you like, and then the molecules react, forming permanent bonds that keep the shape of the mold. Because the starting materials are small molecules, they can fill up all the little features of the mold before it reacts (something that can be difficult with the larger molecule thermoplastics).
As a result of this, thermosets often find uses in polymer/fiber composites, where the small molecules fill molds that contain long thin fibers (typically a few microns thick). The long fibers provide most of the strength, but the thermoset keeps the fibers in place and distributes the force among the fibers.
Slide 18 - The top left image is a close-up of a thermoset matrix surrounding fibers in a thermoset/fiber composite. The fibers are typically on the order of 1 micron in diameter so you can see that the thermoset precursors, the small molecules that will eventually react, are useful to fill those small spaces. The Koenigsegg super car (right image) is a really cool example of such an application, in which the entire car body is composed of a thermoset/carbon fiber composite, desired for its low weight and high strength. So how is the thermoset portion made? Can you control the properties of that portion?
Slide 19 - The thermoset materials undergo a chemical reaction in which new covalent bonds are created. Let's take a look at some of the details. To make a linear molecule (thermoplastic), you mix molecule A (red) and molecule B (blue) together to react. One red group reacts with one blue group to make a purple group. No matter how much A and B react, they always create a linear molecule (imagine many A and B molecules are mixed together).
Next, let's consider the foundation for a thermoset system. Here we have a small molecule A and a small molecule D. They react and form molecule E. The more they react, they form this huge branched interconnected 3-D network (unfortunately, we can only represent 2D on the slides). So how do we control the properties?
Slide 20 - Here we have two bridges. All things being equal, you could probably say that the bridge on the bottom would be more mechanically robust than the bridge on the top. The obvious difference is that the number of connections is different. We consider the bridge with more connections to be stronger.
Slide 21 - Now let's apply that to thermoset. We already talked about the basics of the reaction, but how can we control the strength of our bridge? Let's assume that we have 1,000 molecules: 500 are A and 500 are B, and we make changes as shown on the next slide.
Slide 22 - If we exchange 1 A molecule with 1 D molecule (499A + 500B + 1 D), we have a polymer that is mostly linear, but has one branch at the D molecule.
Is this a strong bridge? (Answer: No)
Slide 23 - If we exchange 10 A molecules with 10 D molecules (490A + 500B + 10 D), we have a polymer that is mostly linear, but now has 10 crosslinks in it at the D molecules.
Is this a strong bridge? (Answer: Not really, but it is getting better.)
Slide 24 - What if we exchange the 500 A molecules with 333 D molecules? Every A functional group can react with a D functional group. You have optimized the number of connections by achieving stoichiometry—or establishing relative quantities.
Is this a strong bridge? (Answer: Very strong. You have the most number of connections possible.)
Slide 25 - What if you keep adding D molecules? Say you exchange 500 A with 10,000 D molecules.
Does that help your strength? Why or why not? (Answer: No, it does not help the strength because you are creating loose ends that do not participate in the connections, but add length to the bridge.)
How would you make a flexible chemical bridge? (Answer: Bridges with fewer chemical connections are more flexible.)
How would you make a strong chemical bridge? (Answer: Bridges with more chemical connections are stronger.)
Does an optimum ratio exist? (Answer: Yes, typically stoichiometry leads to superior mechanical properties.)
Slide 26 – Let's consider a real-life example: we have an epoxy-based molecule and amine-based molecule. The amine can attack the epoxy forming crosslinks. Each hydrogen on the nitrogen is referred to as an active hydrogen, meaning that it can participate in the reaction. The amine here has six active hydrogens, so it has a functionality of six, while the epoxy has two epoxide groups. Following the same ideas as before, we can vary the properties by varying the ratio of amine and epoxy, which is exactly what we are going to explore in the associated activity.
Associated Activities
- Bridging to Polymers: Thermoset Lab - Students apply the knowledge they gain in this lesson to optimizing and mechanically testing their own thermosets.
Vocabulary/Definitions
amine: Nitrogen-containing organic compounds that are derived from ammonia.
enthalpic interactions: Attraction and repulsions due to molecular forces, such as Van der Waals.
entropy: The amount of disorder in the system; can be used to discuss how many configurations a polymer chain can take.
epoxy: A molecule that contains an epoxide chemical group; typically used in thermoset.
hardener: A molecule that chemically reacts with the epoxide group on the epoxy to create a new covalent bond. Typically an amine or anhydride based molecule.
Newtonian fluid: A simple fluid in which the state of stress at any point is proportional to the time rate of strain at that point; the proportionality factor is the viscosity coefficient.
non-Newtonian fluid: A viscous fluid whose stress versus strain rate is non-linear. Example: polymer.
physical entanglement: Entanglements at the molecular level that are not due to chemical bonds. A bowl of spaghetti, and how the individual pieces of pasta wrap around each other, is a good analogy of physical entanglement.
polymer: (poly)=many and (mer) = unit. A long chain of covalently bond atoms, primarily composed of carbon-carbon bonds in the backbone of the chain.
polymer chemistry: Organic chemistry concepts that are used to construct polymers.
polymer physics: Physics that govern the inter-/intra-actions of polymer chains.
shear thickening: The increasing of viscosity due to applied shear.
shear thinning: The decreasing of viscosity due to applied shear.
thermoplastic: A polymer system that consists of many linear polymer chains that are only held together by enthalpic interactions and physical entanglements (for example, a bowl of spaghetti).
thermoset: A polymer system that has gone through a curing reaction and is "set" in its final shape; a thermoset cannot be reshaped by heating.
viscosity: A measure of a materials resistance to flow; for example, honey is more viscous than water.
Assessment
Pre-Lesson Assessment
Questions: Before beginning the lesson, lead a discussion by asking students the following questions to gauge (and refresh) their understanding of some basic chemistry concepts.
- What is a polymer? (Answer: A polymer is a long chain of repeating chemical units. The etymology of the word has a similar meaning [poly] = many and [mer] = unit.)
- What are covalent bonds? (Answer: Chemical bonds that share electrons.)
- Within the context of molecular interaction, what are physical interactions? (Answer: Physical interactions between molecules are those that arise from forces other than covalent bonds, such as Van der Waals, hydrogen bonds and electrostatic interactions. They can be more easily broken than covalent bonds.)
- What is viscosity? (Answer: A measure of a material's resistance to flow; for example, honey is more viscous than water.)
Embedded Assessment
Questions: Throughout the course of the lesson and PowerPoint® presentation, ask students the embedded questions to check for understanding. Questions are included on slides 3-8, 10-15, 19, 21, 22 and 31-35. Engage all students in answering the questions in order to assess their comprehension while delivering the lesson content.
Post-Lesson Assessment
Worksheet: Review students' answers to the 19 questions in the Close Encounters Worksheet to assess their understanding of the lesson's content. The questions are a review of the information taught during the presentation. Answers are provided on the Close Encounters Worksheet – Answer Key.
Additional Multimedia Support
Three Visceoelastic Effects in the One Liquid video (1:06 minutes) shows the Barus Effect (extrudate swell), Weissenberg Effect (rod-climbing) and Kaye Effect (liquid leaping lanyards): https://www.youtube.com/watch?v=nX6GxoiCneY
Defying Gravity video (6 seconds): https://www.youtube.com/watch?v=w0Ib25BPR2I
Slime - Polyvinyl Alcohol with Borax demonstration instructions: http://ncsu.edu/project/chemistrydemos/Organic/Slime.pdf
Subscribe
Get the inside scoop on all things TeachEngineering such as new site features, curriculum updates, video releases, and more by signing up for our newsletter!More Curriculum Like This

Students act as engineers to learn about the strengths of various epoxy-amine mixtures and observe the unique characteristics of different mixtures of epoxies and hardeners. Student groups make and optimize thermosets by combining two chemicals in exacting ratios to fabricate the strongest and/or mo...

Students explore the chemical identities of polymeric materials frequently used in their everyday lives. They learn how chemical composition affects the physical properties of the materials that they encounter and use frequently, as well as how cross-linking affects the properties of polymeric mater...

Students are challenged to think as biomedical engineers and brainstorm ways to administer medication to a patient who is unable to swallow. They learn about the advantages and disadvantages of current drug delivery methods—oral, injection, topical, inhalation and suppository—and pharmaceutical desi...

Students learn about the basics of molecules and how they interact with each other. They learn about the idea of polar and non-polar molecules and how they act with other fluids and surfaces. Students acquire a conceptual understanding of surfactant molecules and how they work on a molecular level. ...
References
Colby, R. H., Boris, D. C., Krause, W. E., Dou, S. 2007. Shear thinning of unentangled flexible polymer liquids. Rheol Acta 46, pp. 569–575.
Hiemenz, Paul and Lodge, Timothy. Polymer Chemistry. Boca Raton, FL: CRC Press, 2007.
Copyright
© 2013 by Regents of the University of Colorado; original © 2012 University of HoustonContributors
Brian Rohde; Don McGowanSupporting Program
National Science Foundation GK-12 and Research Experience for Teachers (RET) Programs, University of HoustonAcknowledgements
This digital library content was developed by the University of Houston's College of Engineering under National Science Foundation GK-12 grant number DGE-0840889. However, these contents do not necessarily represent the policies of the NSF and you should not assume endorsement by the federal government.
Last modified: May 30, 2019
User Comments & Tips