Quick Look
Grade Level: 6 (5-7)
Time Required: 15 minutes
Lesson Dependency: None
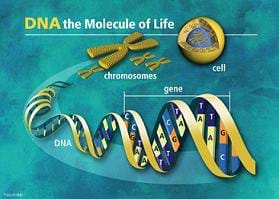
Summary
As a class, students work through an example showing how DNA provides the "recipe" for making human body proteins. They see how the pattern of nucleotide bases (adenine, thymine, guanine, cytosine) forms the double helix ladder shape of DNA, and serves as the code for the steps required to make genes. Students extend their knowledge by conducting the associated activities to learn ways that engineers and scientists are applying their understanding of DNA in our world.Engineering Connection
Once it was discovered that DNA contains the instructions for making cells and proteins, the way was open for many diverse engineering applications. Genetic engineers find ways to modify the DNA of organisms to create a desired trait or protein, such as making a crop resistant to a certain herbicide or making bacteria that create human insulin. Forensic engineers use DNA evidence to help determine people's innocence or guilt of crimes. Agricultural engineers create genetically-modified crops to enable the cultivation of food in areas poorly suited for farming.
Learning Objectives
After this lesson, students should be able to:
- Describe DNA (deoxyribonucleic acid).
- Describe ways in which engineers are involved with genetics and the human body.
Introduction/Motivation
If you want to bake a batch of chocolate chip cookies, what would you need? You probably need some chocolate chips, flour, sugar, butter and eggs. And maybe some nuts or rolled oats if you like. But, how do you know how to make it? By using a recipe! A recipe contains the instructions for how to put all the ingredients together to make the cookies.
Now let's say instead of making chocolate chip cookies, you wanted to make a person! What would you need? (Write on the board an "ingredient list" generated by the students; it might contain: a father [sperm], a mother [egg], uterus, food, proteins, bones, muscles, organs, air, water, elements, minerals, etc.) (After the list is created, ask the students the following question.) And, where is the recipe that tells us how to combine all the ingredients to make a human? (Take suggestions from the students.) That's right, our DNA contains the recipe for making the cells and proteins in our bodies.
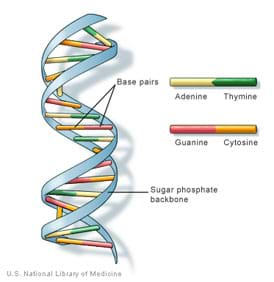
DNA stands for deoxyribonucleic acid. DNA is a pair of molecules usually found in each cell's nucleus that contains codes (the recipe) for the proteins that make our body tissues (show students Figure 1 or a similar DNA representation).
DNA is made of two long molecules that are hydrogen-bonded between the base pairs. (The hydrogen bond is an intermolecular force induced by the pulling away of hydrogen's electron by one base [giving hydrogen a positive charge] and the negative charge of the other base nearby). The base pairs—adenine (A) bonding with thymine (T), and cytosine (C) bonding with guanine (G)—form a code down the length of the DNA.
The order of the nucleotide bases forms a code that specifies which proteins our cells make, and consequently tells our cells what to do. Imagine untwisting the DNA (see Figure 1) and spreading it out flat so that we can see the order of the nucleotide bases. Let's write down the order of the nucleotide bases as an example. (Write the following on the board.)

Notice how the nucleotide bases are matched to form base pairs. These pairs are the actual recipe for making proteins. (To teach the students more about making proteins, or protein synthesis, see the example in the Lesson Background section.)
Now that you understand that DNA contains instructions, let's talk about genes. A gene is a segment of DNA that gets translated into a protein. Genes are more commonly thought of as units for heredity, such as the gene for brown eyes or the genes responsible for being tall or having high blood pressure. DNA, and consequently genes, is passed from a father to his offspring through his sperm, and from a mother to her offspring through her egg. Children have a 50-50 mix of DNA from their parents. Humans have about 25,000 genes. Any two people, though they look different and may come from opposite ends of the world, are 99.9% similar in their genes. Our genetic make up is 98% the same as chimpanzees, 92% the same as mice, and 18% the same as weeds. Clearly, tiny differences in our genes can cause huge physical differences!
Our growing understanding of genetics has led to many diverse engineering applications, including ways to improve crops, catch criminals, detect diseases and determine paternity and ancestry. For many different applications, engineers design DNA collection and analysis tools, equipment and processes. Biomedical engineers specializing in genetics find ways to modify the DNA of organisms to create a desired trait or protein (such as making bacteria that create human insulin).
Just as children inherit their hair or eye color from their parents, they can also inherit genetic diseases. DNA medical analysis has provided the ability to pinpoint the cause of human genetic disease and detect individuals who are predisposed to inherited diseases (such as genetic liver disease). Modern medicine cannot prevent inherited diseases, but can often improve a person's health and life expectancy through engineered treatments.
DNA breakthroughs have led to engineered techniques and tools to rapidly detect and identify outbreaks of infectious diseases, such as severe acute respiratory syndrome (SARS virus). Biomedical engineers help public health practitioners track down the source of infectious disease outbreaks, whether from natural or human sources, which saves many lives. Refer to the DNA Profiling & CODIS: Who Robbed the Bank? activity to have students use DNA profiles to have crack mystery cases! Then have students expand their knowledge with the DNA Build activity by building DNA models to investigate codes relating to certain physical characteristics such as eye and hair color.
Lesson Background and Concepts for Teachers
The Study of Genetics
Gregor Mendel (1822-84) was the first scientist to study genetics through the study of peas. He used pea plants because they were easy to cross-pollinate and fast to see changes in the plants. He studied certain characteristics of pea plants, such as height. He noticed that by cross-pollinating short and tall plants, he could get a certain number of tall plants and a certain number of small plants, depending on the generation. In comparing his study of peas to humans, he found that each pea received its traits from its parent plants. Mendel came up with principles that still hold true today.
The Science of Genetics
Cells are in all living things, and contained within each cell is a nucleus. Within the nucleus is genetic information, in the form of chromosomes, which make up DNA strands. Forty-six chromosomes make up the average human cell. Twenty-three chromosomes have 23 pairs. Red blood cells, sperm and eggs are the only cells in the human body that do not have 46. In human reproduction, a sperm joins an ovum to create a zygote, which has chromosomes made of genes that decide what the baby will look like.
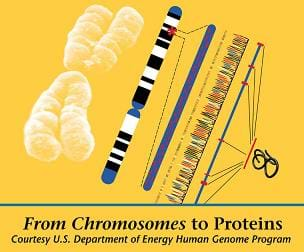
Protein is an important component in the body; it is the building block for everything in the body. Protein is made from the "recipe" contained in the gene. A gene is made from DNA. DNA is arranged in a special pattern that has the recipe contained within in it to make the genes. Each DNA strand is unique to the individual from where it resides. The recipe is known as DNA code. Some people picture a DNA strand as a ladder, with each rung of the ladder made from a pair of DNA letters representing the nucleotide bases. The letters are A, T, G and C, with A only binding to T, and G only binding to C. The binding of these letters forms base pair bonds in a double helix shape.
Protein Synthesis
Protein synthesis is how a piece of DNA provides a code for how to make proteins. In the cell's nucleus, a protein called RNA (ribonucleic acid) polymerase attaches to the DNA strand, stretches it out and separates a section of it:

Now, the RNA polymerase travels down this strand and uses these bases as a template for creating mRNA (messenger RNA). It is called messenger RNA because it carries the message coded by these bases to the ribosomes outside the cell nucleus.

Note that the mRNA is a copy of DNA strand 2 except that uracil (U), another nucleotide base, replaces thymine (T) in mRNA. As the mRNA is made, the RNA polymerase reattaches DNA strand 1 and DNA strand 2.
The mRNA travels outside the cell's nucleus into the cell's cytoplasm and then attaches to ribosomes, which are proteins that can be thought of as cells' protein factories. The ribosome reads the mRNA in sets of three bases, called codons. The codons specify which amino acids, the building blocks of proteins, are made. In our example, a ribosome would travel down the DNA in several steps, making a protein specified by the amino acids called for by each codon:
Step 1: Ribosome reads codon AUG, which means Start Translation (start making protein).

Step 2: Ribosome reads codon UGC, which means make the amino acid cysteine.

Step 3: Ribosome reads codon CUC, which means make the amino acid leucine.

Step 4: Ribosome reads codon UAG, which means End Translation.

(See a complete list of codons and the amino acids they specify in the Wikipedia RNA codon table at http://en.wikipedia.org/wiki/Codon.)
The protein made in our example does not really exist; it just illustrates how DNA contains the codes necessary for protein synthesis and how protein synthesis is carried out. An example of a real protein coded for by DNA is the hormone insulin, which controls the concentration of glucose in our blood. Insulin is made of 51 amino acids.
To summarize, DNA contains nucleotide base codes that are transcribed by RNA polymerase to make mRNA, which takes the code from the nucleus of the cell to the ribosomes in the cytoplasm. The ribosomes translate the codons in the mRNA to make amino acid chains that become proteins. These proteins regulate how our body functions and how we develop.
Lesson Closure
Tell me what you know about DNA. (Expected answers: DNA is found in the nucleus of our cells. It is like a recipe. It is shaped like a double-helix ladder. The backbone is made of deoxyribose, a sugar phosphate, and the ladder rungs are the nucleotide bases. The nucleotide bases are the codes for the proteins that our cells make. A gene is a segment of DNA that gets translated into a protein.)
What are the ways that scientists and engineers are using our understanding of DNA? (Possible answers: To detect inherited diseases in people; to identify infectious diseases and track down their sources; for diagnosis, treatment and [possibly] prevention of human diseases such as cancer, cystic fibrosis and heart disease; to modify organisms for a desired trait or protein; to improve crops; to catch criminals; to examine DNA as part of crime, paternity and ancestry investigations.)
Vocabulary/Definitions
biomedical engineering: The application of engineering techniques to the understanding of biological systems and the development of therapeutic technologies and devices. Kidney dialysis, pacemakers, synthetic skin, artificial joints, and prostheses are some products of biomedical engineering. Also called bioengineering.
DNA: Deoxyribonucleic acid contains the genetic instructions that control the biological development of our cells and the proteins the cells make. DNA codes the sequence of the amino acids in proteins using the genetic code, a triplet code of nucleotide bases.
engineer: A person who applies his/her understanding of science and math to creating things for the benefit of humanity and our world.
gene: Segments of DNA that get translated into proteins.
nucleotide base: The part of RNA and DNA involved in pairing; it includes cytosine, guanine, adenine, thymine (DNA) and uracil (RNA), abbreviated as C, G, A, T and U. They are usually simply called bases in genetics. Also called base pairs.
protein synthesis: The process by which proteins are made. DNA nucleotide base codes are transcribed by RNA polymerase to make mRNA, which takes the code from the nucleus of the cell to the ribosomes in the cytoplasm. The ribosomes translate the codons in the mRNA to make amino acid chains that become proteins.
ribosome: A tiny, round particle composed of RNA and protein that is found in the cytoplasm of living cells and serves as the site of assembly for polypeptides encoded by messenger RNA.
Assessment
Pre-Lesson Assessment
Discussion Question: Ask students to write a "recipe" for what it takes to make a human. Compare lists. Lead into a discussion of DNA and how it contains the "recipe" for our body.
Post-Introduction Assessment
Question/Answer: Ask students questions and have them raise their hands to respond. Write their answers on the board.
- Where is the information stored for how to make our cells? (Answer: In our DNA, which stands for deoxyribonucleic acid.)
- What is a gene? (Answer: A gene is a segment of DNA that gets translated into a protein.)
- What are the ways scientists and engineers are using our understanding of DNA to benefit society? (Possible answers: To detect inherited diseases in people; to identify infectious diseases and track down their sources; for diagnosis, treatment and [possibly] prevention of human diseases such as cancer, cystic fibrosis and heart disease; to modify organisms for a desired trait or protein; to improve crops; to catch criminals; to examine DNA as part of crime, paternity and ancestry investigations.)
Lesson Summary Assessment
Engineering Impacts: We have learned that biomedical engineers develop the tools and processes to make possible DNA analysis to modify and improve crops, catch criminals, detect diseases and determine paternity and ancestry. Biomedical engineers have even found ways to modify the DNA of organisms to create a desired trait or protein (such as making bacteria that create human insulin). Have students think about the potential abstract impacts of DNA engineering on society and the environment. Then assign students to choose one possible impact area (such as environmental, ethical, economical, societal [people]) and write a persuasive argument paragraph describing the possible impacts (positive and negative) on that area due to the engineering of DNA.
Lesson Extension Activities
Research & Report: Have students research the "human genome project" to find out what it is and why it is significant. Report back to the class on five important results from this research project. Start your research at Oak Ridge National Laboratory's About the Human Genome Project website: http://www.ornl.gov/sci/techresources/Human_Genome/project/about.shtml
Have students research genetically-modified foods, reporting to the class on whether they pose a health and/or environmental risk. In the laboratory, foods are genetically modified, selecting for desired characteristics. For example, in 1992 the FlavrSavr® tomato was created by modifying its DNA so that the tomato resists rotting. What about the modified fruits at the grocery store, advertised that they do not have seeds? What are the benefits? What are the controversies? Start your research at Oak Ridge National Laboratory's Genetically Modified Foods and Organisms website: http://www.ornl.gov/sci/techresources/Human_Genome/elsi/gmfood.shtml
Have students learn more about protein sysnthesis. Ask them to describe how a protein gets made, starting from its nucleobase code in the DNA. (Answer: The nucleotide base codes are transcribed by RNA polymerase to make mRNA, which takes the code from the nucleus of the cell to the ribosomes in the cytoplasm. The ribosomes translate the codons in the mRNA to make amino acid chains that become proteins.)
Additional Multimedia Support
Use interactive multimedia activities to explore some of the applications of DNA technology at Koshland Science Museum's Putting DNA to Work website: https://koshland-science-museum.org/exhibitdna/inf02.jsp?PF=1#.T_tOjFJ6DqM
Excellent DNA graphics and images suitable for PowerPoint presentations or color overhead transparences are available at the US Department of Energy's Human Genome Program's image gallery website: http://genomics.energy.gov/gallery/
Subscribe
Get the inside scoop on all things TeachEngineering such as new site features, curriculum updates, video releases, and more by signing up for our newsletter!References
Blumberg, Robert B. (ed.) MendelWeb. Edition 97.1 (February 22, 1997). MendelWeb.
Gene. Last updated February 24, 2009. Wikipedia, The Free Encyclopedia. Accessed February 24, 2009. http://en.wikipedia.org/wiki/Genes
Genetic code. Last updated February 21, 2009. Wikipedia, The Free Encyclopedia. Wikimedia Foundation, Inc. Accessed February 24, 2009. (Includes a complete list of codons and the amino acids they specify in the RNA codon table) http://en.wikipedia.org/wiki/Codons
Genome Programs of the US Department of Energy. US Department of Energy. (Good resource for information and images)
Protein biosynthesis. Last updated January 16, 2009. Wikipedia, The Free Encyclopedia. Accessed February 24, 2009. http://en.wikipedia.org/wiki/Protein_biosynthesis
Copyright
© 2007 by Regents of the University of ColoradoContributors
Frank Burkholder; Jessica Todd; Malinda Schaefer Zarske; Denise W. CarlsonSupporting Program
Integrated Teaching and Learning Program, College of Engineering, University of Colorado BoulderAcknowledgements
The contents of this digital library curriculum were developed under grants from the Fund for the Improvement of Postsecondary Education (FIPSE), U.S. Department of Education and National Science Foundation (GK-12 grant no. 0338326). However, these contents do not necessarily represent the policies of the DOE and NSF, and you should not assume endorsement by the federal government.
Last modified: September 6, 2024
User Comments & Tips