Quick Look
Grade Level: 11 (10-12)
Time Required: 1 hours 15 minutes
Expendable Cost/Group: US $7.00 Two ways to conduct this activity: Option 1 has no expendables cost but requires the use of materials not available for public purchase. Option 2 uses common materials with an expendable cost of $7. See the Materials List for details.
Group Size: 3
Activity Dependency:
Subject Areas: Physical Science, Physics, Problem Solving, Science and Technology
NGSS Performance Expectations:
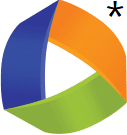
HS-ETS1-1 |
HS-PS4-1 |
MS-PS4-2 |
Summary
In the first half of this two-part activity, students practice solving problems involving refraction using the index of refraction and Snell's law equations; they mathematically solve for precise angles and speeds caused by refraction. In the second half of the activity, a hands-on lab, they apply the analytical skills required by the problem set to reflectance measurements of porous silicon thin films, including how reflectance measurements would change if various aspects of the film were altered. Students predict the data output in the form of reflectance measurements when samples are altered, which connects to the idea of being able to make predictions about the data output of a biosensing thin film that couples with a target molecule.Engineering Connection
In order to create workable products, engineers need more than basic information about physical concepts and how to manipulate them to perform tasks. More specifically, the information must be quantified or measurable in numerical form through precise analysis in order to ensure that exact inputs produce exactly the same outputs every time a task is performed. In this activity, students tackle this aspect of engineering as they solve problems for precise angles and speeds, and predict data output (reflectance measurement) when samples are altered.
Learning Objectives
After this activity, students should be able to:
- Accurately solve a refraction problem for a missing angle or index of refraction.
- Explain in at least two complete sentences how connection with a target molecule alters the data output of a porous silicon thin film.
Educational Standards
Each TeachEngineering lesson or activity is correlated to one or more K-12 science,
technology, engineering or math (STEM) educational standards.
All 100,000+ K-12 STEM standards covered in TeachEngineering are collected, maintained and packaged by the Achievement Standards Network (ASN),
a project of D2L (www.achievementstandards.org).
In the ASN, standards are hierarchically structured: first by source; e.g., by state; within source by type; e.g., science or mathematics;
within type by subtype, then by grade, etc.
Each TeachEngineering lesson or activity is correlated to one or more K-12 science, technology, engineering or math (STEM) educational standards.
All 100,000+ K-12 STEM standards covered in TeachEngineering are collected, maintained and packaged by the Achievement Standards Network (ASN), a project of D2L (www.achievementstandards.org).
In the ASN, standards are hierarchically structured: first by source; e.g., by state; within source by type; e.g., science or mathematics; within type by subtype, then by grade, etc.
NGSS: Next Generation Science Standards - Science
NGSS Performance Expectation | ||
---|---|---|
HS-ETS1-1. Analyze a major global challenge to specify qualitative and quantitative criteria and constraints for solutions that account for societal needs and wants. (Grades 9 - 12) Do you agree with this alignment? |
||
Click to view other curriculum aligned to this Performance Expectation | ||
This activity focuses on the following Three Dimensional Learning aspects of NGSS: | ||
Science & Engineering Practices | Disciplinary Core Ideas | Crosscutting Concepts |
Analyze complex real-world problems by specifying criteria and constraints for successful solutions. Alignment agreement: | Criteria and constraints also include satisfying any requirements set by society, such as taking issues of risk mitigation into account, and they should be quantified to the extent possible and stated in such a way that one can tell if a given design meets them. Alignment agreement: Humanity faces major global challenges today, such as the need for supplies of clean water and food or for energy sources that minimize pollution, which can be addressed through engineering. These global challenges also may have manifestations in local communities.Alignment agreement: | New technologies can have deep impacts on society and the environment, including some that were not anticipated. Analysis of costs and benefits is a critical aspect of decisions about technology. Alignment agreement: |
NGSS Performance Expectation | ||
---|---|---|
HS-PS4-1. Use mathematical representations to support a claim regarding relationships among the frequency, wavelength, and speed of waves traveling in various media. (Grades 9 - 12) Do you agree with this alignment? |
||
Click to view other curriculum aligned to this Performance Expectation | ||
This activity focuses on the following Three Dimensional Learning aspects of NGSS: | ||
Science & Engineering Practices | Disciplinary Core Ideas | Crosscutting Concepts |
Use mathematical representations of phenomena or design solutions to describe and/or support claims and/or explanations. Alignment agreement: | The wavelength and frequency of a wave are related to one another by the speed of travel of the wave, which depends on the type of wave and the medium through which it is passing. Alignment agreement: | Empirical evidence is required to differentiate between cause and correlation and make claims about specific causes and effects. Alignment agreement: |
NGSS Performance Expectation | ||
---|---|---|
MS-PS4-2. Develop and use a model to describe that waves are reflected, absorbed, or transmitted through various materials. (Grades 6 - 8) Do you agree with this alignment? |
||
Click to view other curriculum aligned to this Performance Expectation | ||
This activity focuses on the following Three Dimensional Learning aspects of NGSS: | ||
Science & Engineering Practices | Disciplinary Core Ideas | Crosscutting Concepts |
Develop and use a model to describe phenomena. Alignment agreement: | A sound wave needs a medium through which it is transmitted. Alignment agreement: When light shines on an object, it is reflected, absorbed, or transmitted through the object, depending on the object's material and the frequency (color) of the light.Alignment agreement: The path that light travels can be traced as straight lines, except at surfaces between different transparent materials (e.g., air and water, air and glass) where the light path bends.Alignment agreement: A wave model of light is useful for explaining brightness, color, and the frequency-dependent bending of light at a surface between media.Alignment agreement: However, because light can travel through space, it cannot be a matter wave, like sound or water waves.Alignment agreement: | Structures can be designed to serve particular functions by taking into account properties of different materials, and how materials can be shaped and used. Alignment agreement: |
International Technology and Engineering Educators Association - Technology
-
Optimize a design by addressing desired qualities within criteria and constraints.
(Grades
9 -
12)
More Details
Do you agree with this alignment?
State Standards
Tennessee - Science
-
Investigate reflection, refraction, diffraction, and interference of light waves.
(Grades
9 -
12)
More Details
Do you agree with this alignment?
-
Examine properties of light waves.
(Grades
9 -
12)
More Details
Do you agree with this alignment?
-
Solve problems related to Snell's law [Index of refraction: n = (sin θr / sin θi); Snell's law: ni sin θi = nr sin θr].
(Grades
9 -
12)
More Details
Do you agree with this alignment?
Materials List
Two options are suggested for how to conduct this activity. Option 1—When Silicon Talks, requires a silicon thin film, which is not available for public purchase, and is likely only accessible through a partnership with a research university. If this material cannot be acquired, follow the alternate Option 2—When Thin Film Talks procedure, which uses more readily available materials.
For Option 1—When Silicon Talks, each group needs:
- a sample single-layer porous silicon thin film, which is not available for public purchase but may be available by donation from universities with photonics laboratories; contact physics, electrical engineering and/or chemistry departments at any major university to inquire; if it is not possible to acquire this material, gather the Option 2 materials and follow the Option 2 procedure instead
- Measuring Refraction: Silicon Worksheet, one per student
For Option 1—When Silicon Talks, to share with the entire class:
- (optional) Ocean Optics Spectrometer that is capable of measuring reflectance in the visible range; a complete kit including spectrometer, light source and fiber optic cable is ~$4,000 new; used systems may be available for less; specific pricing varies since kits are assembled individually; contact Ocean Optics service representatives listed at http://www.oceanoptics.com/contact/
- (optional) computer or laptop with SpectraSuite® software installed, and a USB cable to the spectrometer; a modular, Java-based spectroscopy software platform that operates on 32- and 64-bit Windows, Macintosh and Linux operating systems to control any Ocean Optics USB spectrometer; available from Ocean Optics, same details apply as with the spectrometer; old software versions may be available for free upon request
- Alternative: If a spectrometer with computer and software is not available, use the Screen Captures of Spectrometer Images as an overhead projector visual aid and/or group handout
- classroom projector
- tweezers, for handling the silicon samples
- (optional) clamp, to secure the fiber optic cable
For Option 2—When Thin Film Talks, each group needs:
- 4 pieces black construction paper
- 1 disposable plastic or aluminum tray, such as a disposable litter tray for $3.28 each at Walmart
- 1 bottle clear nail polish, such as Sally Hansen brand for $2.97 per bottle at Walmart
- 1 plastic transfer pipet, such as 3-ml graduated plastic transfer pipet, 20 for $4.76 at https://www.amazon.com/Rienar-Disposable-Transfer-Graduated-Pipettes/dp/B00P7QZDK4/ref=sr_1_1?ie=UTF8&qid=1512079406&sr=8-1&keywords=3-ml+graduated+plastic+transfer+pipet
- 1 plastic fork
- 4 paper towels
- personal protective equipment: gloves, goggles, well-ventilated lab area
- Measuring Refraction: Thin Films Worksheet, one per student
- classroom projector
- access to water
Worksheets and Attachments
Visit [www.teachengineering.org/activities/view/van_oddsofcancer_lesson03_activity1] to print or download.Pre-Req Knowledge
Students must have:
- A basic knowledge of algebra and elementary trigonometry, and be able to solve simple algebraic and trigonometric problems.
- A conceptual understanding of refraction and the challenge problem presented in the first lesson, Tell Me Doc—Will I Get Cancer?
Introduction/Motivation
Now that we know what refraction is, how it works and how we can use refraction to alter the colors we see from a thin film, is it possible to use that information to make a workable device that can sense the presence of specific genes? We already know that, in theory, the answer is yes. But we're not quite there.
The answer to our challenge question is not quite complete. (As necessary, ask students to recall/explain the challenge question as a quick review.) What else is necessary before we can present our findings? (Expect various answers. The best answer is "repeatability.") Yes, we must prove that our theory is correct by producing exact, repeatable results. And if we want exact results, what do we need? (The best answers are "a way to measure" and "an equation/numbers.")
As you probably guessed from previous units, if we want exact measurements, an equation exists that we can apply to get them, and thereby prove that our equation correctly predicts what will happen. That way, if we create a device that can detect certain genes, we can prove that a single result, or output measurement, must come only from the input of the gene we want. That's why this activity comprises the Test Your Mettle phase of the Legacy Cycle. You are testing what you know to see if is correct and repeatable before you publish your findings.
So, what are these equations, and how do we use them? Well, that's what this activity is all about. I'll help you get started because this may be unfamiliar to you at first, but you'll find very shortly that it makes sense. Let's begin!
Procedure
Background
This first half of this activity is primarily focused on practice using the equations for index of refraction and Snell's law, which are introduced and explained in the associated lesson.
As explained in the Materials List, the second half of the activity may be conducted in two ways, depending on the availability of materials and equipment. Both ways cover the same objectives. If you are following Option 1—When Silicon Talks, note that the second portion of the activity involves using a laptop and spectrometer to measure reflectance of thin films. If these resources are not available, use the Screen Captures of Spectrometer Images (also Figures 1-3) as a substitute. This means that in the Option 1 Procedure-With the Students, groups would interpret the provided images rather than use the equipment to generate their own graphs. The alternate procedure, Option 2—When Thin Film Talks, uses common materials that may be more accessible. If materials are available for both options, feel free to have students conduct both procedures to reinforce the concepts.
During the activity, be sure to emphasize how a change in refractive index (caused by the presence of a substance in the porous film, or the binding of a target molecule to the molecule in your film) causes a shift in wavelengths of light that get reflected, which is observable and measurable data output. The way this works is if we take a reflectance measurement using a spectrometer, we get a sinusoidal wave pattern when viewing percentage of light reflected vs. wavelength. This is what we would expect, since the pores cause specific wavelengths of light to interfere constructively (aka, an interference pattern). But if the index of refraction of the film changes, we expect to see different wavelengths interfering constructively (see detailed explanation in the lesson 2 of the unit). This means that the sinusoidal pattern on the graph would "shift" left or right to indicate that longer or shorter wavelengths are interfering constructively as they are refracted. Snell's law confirms this by indicating how a change in "n" creates a change in "θ" to produce different constructively interfered wavelengths.
Option 1—When Silicon Talks: Before the Activity
- Make copies of the Measuring Refraction: Silicon Worksheet, one per student.
- Place a silicon sample at each lab station.
- Set up the spectrometer with a USB cable attached to a computer or laptop, and the SpectraSuite® software opened. See the Troubleshooting Tips section for how to set up the equipment.
- If a spectrometer is not being used, have students use the Screen Captures of Spectrometer Images (same as Figure 1-3) instead. Display one of these reflectance images on a projector screen, but keep it hidden or muted until it is time to show the students.
Option 1—When Silicon Talks: With the Students
- Divide the class into groups of three students each and have them complete page 1 of the silicon worksheet (six word problems). Circulate the room to monitor progress and answer questions. Below are alternate methods for completing this portion of the activity:
Method 1: Assign one problem to each group. Then have each group present its solution to the class, while the class checks the work and writes down the solution.
Method 2: Have the class complete each problem one-by-one on small whiteboards (or on sheet protectors covering white paper) to hold up for the teacher to check as they complete them. Once everyone has the correct solution, move on to the next problem. This method adapts well to a competition, with students racing to be the first to solve each problem correctly.
- While students are working on the worksheet problem set, have groups rotate to the laptop/spectrometer setup, and place a sample under the detector using tweezers. Expect graphs to be similar to Figure 1; save the graphs that appear. If you do not have this equipment available, skip this step.
- As students finish the problem set, direct their attention to a reflectance graph projected in the classroom, and have them begin to answer the questions on worksheet page 2 (questions 1-5).
- When everyone has finished the problem set, work through the When Biosensors Talk set of problems on worksheet page 2 as a class. If you do not have access to the spectrometer equipment, use the Screen Captures of Spectrometer Images (also Figures 1–3). If you do have the equipment, display the images that students saved from the samples, asking students to note the differences between them. Emphasize that different wavelengths would show a higher percentage of reflectance if the index of refraction of the film is different (as it would be if a target molecule, such as DNA, bonded with your particular sample). This corresponds to a shift in the sinusoidal wave pattern.
Figure 1. An example interference pattern produced when a reflectance measurement of a porous silicon film is taken. Figure 2. An example interference pattern produced when a reflectance measurement of a porous silicon film is taken. Figure 3. An example interference pattern produced when a reflectance measurement of a porous silicon film is taken. - Conclude the activity by having students individually solve the worksheet final problem, and turn in their worksheets for grading. Conclude the lesson by assigning students to answer the two questions provided in the associated lesson's Assessment section.
Option 2—When Thin Film Talks: Before the Activity
- Make copies of the Measuring Refraction: Thin Films Worksheet, one per student.
- At each group lab station, place four pieces of black construction paper, disposable tray, plastic fork, plastic transfer pipet and bottle of clear nail polish.
- Make copies of the Nail Polish Thin Film Procedure Handout (two instruction sets per sheet; also presented below), and place a copy at each lab station.
- Do a trial run creating a nail polish thin film since variations in nail polish and paper may affect the outcome. This gives you a better understanding of how to successfully get the films onto the paper, as well as expected drying time (estimated 10-15 minutes).
Option 2—When Thin Film Talks: With the Students
- Divide the class into groups of three student each and have them complete page 1 of the thin films worksheet (six word problems). Circulate the room to monitor progress and answer questions. Below are alternate methods for completing this portion of the activity:
Method 1: Assign one problem to each group. Then have each group present its solution to the class, while the class checks the work and writes down the solution.
Method 2: Have the class complete each problem one-by-one on small whiteboards (or on sheet protectors covering white paper) to hold up for the teacher to check as they complete them. Once everyone has the correct solution, move on to the next problem. This method adapts well to a competition, with students racing to be the first to solve each problem correctly.
- While students are working on the worksheet problem set, have groups rotate to the lab stations to create their "nail polish thin films." Direct students to follow the instructions on the nail polish handout (also provided below). Rotate between students working on the problem set and groups creating films to provide feedback and troubleshooting assistance. When students are finished creating their films, have them leave them to dry and return to working on the problem set. An alternate sequencing is to have all students create the films at once, then return to the problem set while the films dry.
Nail Polish Thin Film Procedure
- Put on your lab goggles and gloves. Work in a well-ventilated area.
- Fill the tray 2–3 cm deep with water.
- Nearby, lay out flat a paper towel.
- Submerge the black construction paper in the water. Use the plastic fork to keep it submerged.
- Use the pipet to drop a single drop of clear nail polish onto the water surface. Let the drop fall from about 5–10 cm above the water. Within a few seconds, the nail polish should spread out, creating a film on the water in which rainbow colors can be seen.
- Carefully and smoothly slide the paper out of the water at an angle, capturing the nail polish film on the surface of the paper. Place the paper on a paper towel to dry. Be careful—the films are fragile!
- Repeat this procedure so each student in your group has a thin film. Note that you have an extra piece of paper and paper towel at your station—this is in case of mess-ups. See the teacher if you need more paper or paper towels.
- When students have finished the problem set, and all the films are dry (10–15 minutes drying time), have students look at their films and complete the When Biosensors Talk section on worksheet page 2 (questions 1-5). Monitor their progress and provide feedback. As students finish, direct them to return to their original seats and individually complete the worksheet final problem.
- When everyone has finished the problem set and the When Biosensors Talk lab questions, lead a class discussion to share results; call on students to read their worksheet question responses. Be sure students understand how different colors seen at different angles are the results of differing path lengths for the light to travel, and how bending light at a different angle can be caused by a different index of refraction (connection to the problem set).
- Conclude the activity collecting the completed worksheets. Conclude the lesson by assigning students to answer the two questions provided in the associated lesson's Assessment section.
Vocabulary/Definitions
angle of incidence: The angle at which light enters a different material, measured from the normal, or an imaginary line perpendicular to the surface of the material.
angle of reflection: The angle at which light is bent after entering a material, measured from the normal, or an imaginary line perpendicular to the surface of the material.
reflectance: The percentage of light incident on a sample that is reflected back to a detector.
sinusoidal: Following the shape or pattern of a sin (or cos) wave.
Assessment
Pre-Activity Assessment
Worksheet-Page 1: Have students complete the refraction problem set on the first page of the appropriate worksheet to assess their understanding of concepts presented in the associated lesson as an assessment of readiness for conducting the activity and subsequent reflectance portions of the activity. If 80% or more of the students get the problem(s) correct, they are ready for the activity.
Activity Embedded Assessment
Worksheet Page 2: While students follow the procedure and complete the When Biosensors Talk portion of worksheet page 2, monitor their progress and participation. Ask questions to prompt them to explain what they are working on, what they have observed, how they are answering certain questions or to explain their answers.
Post-Activity Assessment
Worksheet Final Problem: After students answer the final problem questions, collect and review the worksheets for accuracy. The worksheet questions directly correlate to the activity's learning objectives. If at least 80% of the students get at least 85% correct answers, proceed to teach the next lesson in the unit.
Investigating Questions
- What happens to the angle of refraction if the angle of incidence is increased or decreased? (Answer: The angle of refraction is increased or decreased in direct proportion.)
- What happens to the angle of incidence if the index of refraction is increased or decreased? (Answer: The angle of incidence is increased or decreased in direct proportion.)
- What will happen to the index of refraction if I put DNA in my sample and it couples with a target DNA? (Answer: The index of refraction increases.)
- Why are some wavelengths reflected more than others? (Answer: Only certain wavelengths of light are a direct multiple of the path distance that light must take through the film.)
- What will happen to the reflected wavelengths if I change the index of refraction of my sample? (Answer: The reflected wavelengths either "shift" left or right, towards shorter or longer wavelengths.)
Safety Issues
- Option 1—When Silicon Talks: If students handle the silicon samples, make sure they use tweezers to protect the film from damage and themselves from sharp edges.
- Option 2—When Thin Film Talks: When handling the nail polish, have students use personal protective equipment (gloves and goggles) in a well-ventilated area.
Troubleshooting Tips
Option 1—When Silicon Talks: If you are using a spectrometer with porous silicon samples, use the following procedure to take reflectance measurements:
Prepare the equipment before students use it:
- Couple fiber optic cable from light source to open end (where the sample is placed) and back to the spectrometer. The Spectrometer User's Guide explains this process in more detail.
- Connect the USB cable from spectrometer to computer, and open SpectraSuite®.
- Change integration time to 10 ms, and scans to "average to 10."
- Click File > then New > then New Reflection Measurement.
- In the window that pops up, click Next.
- On the next screen, change scans to "average to 10," and integration time to 10 ms > click Next.
- Click the light bulb > then Next.
- Turn off light source > click dark light bulb > then click Finish.
- Turn light source back on, and wait 3 minutes.
When the students use it:
- Use tweezers to place a silicon sample under the open fiber optic cable. It may be helpful to have a clamp to hold the cable in place.
- A wave pattern should appear on the screen (as necessary, minimize other windows in order to see it).
- Click File > Screen Picture > then choose Accept in the dialogue box that pops up. Save it in whatever folder you choose, under student or group names.
Option 2—When Thin Film Talks
- In advance, do a trial run creating a nail polish thin film since variations in the type of nail polish and paper may affect the outcome. This gives you a better understanding of how to successfully get the films onto the paper, as well as expected drying time, etc.
- Shallow water makes it easier to create the films. Excess water often washes the film away or does not allow the film to even touch the paper.
- If students struggle too much with creating their own films, let them use your sample to answer the lab questions.
Activity Scaling
- For lower grades, make the problem set purely conceptual, that is, if the angle of incidence goes up, what happens to angle of refraction?
- For upper grades, require more detailed explanation on all problems, especially the worksheet final problem.
Subscribe
Get the inside scoop on all things TeachEngineering such as new site features, curriculum updates, video releases, and more by signing up for our newsletter!More Curriculum Like This

Students learn the relevant equations for refraction (index of refraction, Snell's law) and how to use them to predict the behavior of light waves in specified scenarios. After a brief review of the concept of refraction (as learned in the previous lesson), the equations along with their units and v...

Students are introduced to the concept of refraction. After making sure they understand the concepts of diffraction and interference, students work collaboratively to explain optical phenomena that cannot be accounted for via these two mechanisms alone.

Through four lessons and three hands-on activities, students learn the concepts of refraction and interference in order to solve an engineering challenge. Students learn about some high-tech materials and delve into the properties of light, including the equations of refraction (index of refraction,...

Students observe wave refraction in different mediums. They trace the path of light waves of a laser entering a medium and exiting the medium. Then they measure the angle of the refracted ray using a protractor to get an understanding of the concepts of how refraction works in different mediums. By...
References
Klein, Stacy S., Harris, Alene H. "A User's Guide to the Legacy Cycle." Journal of Education and Human Development. Vol. 1, No. 1, 2007. http://www.scientificjournals.org/journals2007/articles/1088.pdf
Nave, Carl R. "Refraction of Light." 2012. HyperPhysics, Department of Physics and Astronomy, Georgia State University, Atlanta, GA. Accessed June 26, 2013. (Source of Snell's law explanation, with some adaptation) http://hyperphysics.phy-astr.gsu.edu/hbase/hframe.html
Refractions and Lenses: Problem Set. 1996-2014. Ray Optics: Refraction and Lenses, The Physics Classroom. Accessed June 26, 2013. (Source of worksheet problems, with some adaptation). http://www.physicsclassroom.com/calcpad/refrn/problems.cfm
Copyright
© 2014 by Regents of the University of Colorado; original © 2012 Vanderbilt UniversityContributors
Caleb SwartzSupporting Program
VU Bioengineering RET Program, School of Engineering, Vanderbilt UniversityAcknowledgements
The contents of this digital library curriculum were developed under National Science Foundation RET grant nos. 0338092 and 0742871. However, these contents do not necessarily represent the policies of the National Science Foundation, and you should not assume endorsement by the federal government.
Last modified: March 17, 2018
User Comments & Tips