Quick Look
Grade Level: 11 (10-12)
Time Required: 1 hours 30 minutes
(time for launch, several hours or more for chase and recovery of payload)
Expendable Cost/Group: US $0.00 See Materials List for details. Note: The cost of this activity, which involves launching a high-altitude balloon, is much higher than normal: $200 per group to build the payload; $1500 to launch the balloon. There are alternatives to launching a high-altitude balloon, including in-class experiments and engineering design activities, as well as data analysis using existing data sets, which will be discussed below.
Group Size: 5
Activity Dependency: None
Subject Areas: Computer Science, Data Analysis and Probability, Earth and Space, Measurement, Physical Science, Physics, Problem Solving, Science and Technology
NGSS Performance Expectations:
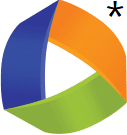
HS-ETS1-1 |
HS-ETS1-2 |
Summary
This activity has students embarking on an ambitious design project: to measure the effect of shielding from cosmic radiation via a high-altitude balloon launch test. Students prepare their payloads for launch, making sure batteries are charged, payloads are complete, flight predictions have been made and approved, etc. On launch day, students launch a latex, high-altitude balloon that carries each space agency’s payload to near space. GPS tracking units follow the balloon flight path in real-time, so the location and altitude of the balloon during flight, when it bursts, and once the payload is on the ground. After landing, participants travel to the landing site and retrieve and examine the payloads.Engineering Connection
Once engineers have a product designed and prototyped, they need to test that the prototype works as expected. To determine whether a design works, engineers need to collect data (here in near space) about whether or not their product (in this case, shielding) works. Engineers can then iterate on future designs based on the data they collect from the tests.
Learning Objectives
After this activity, students should be able to:
- Collect data to test the effectiveness of the radiation shielding device, including a non-shielded Geiger counter for comparison.
- Produce deliverables according to their assigned role (e.g., director presents an overview of the program; economic advisor provides an itemized list and budget; public relations expert produces a logo and poster).
Educational Standards
Each TeachEngineering lesson or activity is correlated to one or more K-12 science,
technology, engineering or math (STEM) educational standards.
All 100,000+ K-12 STEM standards covered in TeachEngineering are collected, maintained and packaged by the Achievement Standards Network (ASN),
a project of D2L (www.achievementstandards.org).
In the ASN, standards are hierarchically structured: first by source; e.g., by state; within source by type; e.g., science or mathematics;
within type by subtype, then by grade, etc.
Each TeachEngineering lesson or activity is correlated to one or more K-12 science, technology, engineering or math (STEM) educational standards.
All 100,000+ K-12 STEM standards covered in TeachEngineering are collected, maintained and packaged by the Achievement Standards Network (ASN), a project of D2L (www.achievementstandards.org).
In the ASN, standards are hierarchically structured: first by source; e.g., by state; within source by type; e.g., science or mathematics; within type by subtype, then by grade, etc.
NGSS: Next Generation Science Standards - Science
NGSS Performance Expectation | ||
---|---|---|
HS-ETS1-1. Analyze a major global challenge to specify qualitative and quantitative criteria and constraints for solutions that account for societal needs and wants. (Grades 9 - 12) Do you agree with this alignment? |
||
Click to view other curriculum aligned to this Performance Expectation | ||
This activity focuses on the following Three Dimensional Learning aspects of NGSS: | ||
Science & Engineering Practices | Disciplinary Core Ideas | Crosscutting Concepts |
Analyze complex real-world problems by specifying criteria and constraints for successful solutions. Alignment agreement: | Criteria and constraints also include satisfying any requirements set by society, such as taking issues of risk mitigation into account, and they should be quantified to the extent possible and stated in such a way that one can tell if a given design meets them. Alignment agreement: Humanity faces major global challenges today, such as the need for supplies of clean water and food or for energy sources that minimize pollution, which can be addressed through engineering. These global challenges also may have manifestations in local communities.Alignment agreement: | New technologies can have deep impacts on society and the environment, including some that were not anticipated. Analysis of costs and benefits is a critical aspect of decisions about technology. Alignment agreement: |
NGSS Performance Expectation | ||
---|---|---|
HS-ETS1-2. Design a solution to a complex real-world problem by breaking it down into smaller, more manageable problems that can be solved through engineering. (Grades 9 - 12) Do you agree with this alignment? |
||
Click to view other curriculum aligned to this Performance Expectation | ||
This activity focuses on the following Three Dimensional Learning aspects of NGSS: | ||
Science & Engineering Practices | Disciplinary Core Ideas | Crosscutting Concepts |
Design a solution to a complex real-world problem, based on scientific knowledge, student-generated sources of evidence, prioritized criteria, and tradeoff considerations. Alignment agreement: Develop a model based on evidence to illustrate the relationships between systems or between components of a system.Alignment agreement: | Criteria may need to be broken down into simpler ones that can be approached systematically, and decisions about the priority of certain criteria over others (trade-offs) may be needed. Alignment agreement: | Models (e.g., physical, mathematical, computer models) can be used to simulate systems and interactions—including energy, matter, and information flows—within and between systems at different scales. Alignment agreement: |
International Technology and Engineering Educators Association - Technology
-
Students will develop an understanding of the relationships among technologies and the connections between technology and other fields of study.
(Grades
K -
12)
More Details
Do you agree with this alignment?
-
Select resources that involve tradeoffs between competing values, such as availability, cost, desirability, and waste, while solving problems.
(Grades
9 -
12)
More Details
Do you agree with this alignment?
-
Demonstrate the use of conceptual, graphical, virtual, mathematical, and physical modeling to identify conflicting considerations before the entire system is developed and to aid in design decision making.
(Grades
9 -
12)
More Details
Do you agree with this alignment?
-
Optimize a design by addressing desired qualities within criteria and constraints.
(Grades
9 -
12)
More Details
Do you agree with this alignment?
State Standards
Wyoming - Science
-
Analyze a major global challenge to specify qualitative and quantitative criteria and constraints for solutions that account for societal needs and wants.
(Grades
9 -
12)
More Details
Do you agree with this alignment?
-
Design a solution to a complex real-world problem by breaking it down into smaller, more manageable problems that can be solved through engineering.
(Grades
9 -
12)
More Details
Do you agree with this alignment?
Materials List
Each group needs:
- One payload completely built and ready to launch. Please refer to the Payload Manual Guide as needed.
- At least one copy of the Payload Manual Guide for the Engineer(s) and Computer Scientist(s) to refer to for directions and guidance building and programming, as well as for other roles to gain an overall idea of the goal for the project.
To share with the entire class:
- Materials to complete a high-altitude balloon launch. In total, this may cost upwards of $1000–$2000. The main components include:
- 2 tanks of Helium ($250–400/tank, each should hold at least 200 cubic ft of helium)
- Compressed gas regulator with hose for filling the balloon ($50, make sure regulator fits the valve on the tank)
- 72-inch parachute ($50–100)
- High-strength (>10lb. strength) string/cord (e.g., kite string, paracord, etc.) to attach payloads to each other and to balloon
- A tracking device that logs GPS location and altitude data during flight and transmits that data back to participants in real-time ($50–500 depending on what is used, see below)
- (Highly recommended) Backup GPS device such as a SPOT GPS transmitter ($200–300 each including the cost of monthly subscription to access satellite network)
- (Optional) Action camera(s) such as a GoPro ($200–400 each)
- Miscellaneous items:
- Large wrench to tighten regulator to helium tank
- Additional string/cord to hold balloon down prior to launch (i.e., safety line),
- Digital fish scale to measure balloon’s free lift
- Zip ties
- Electrical tape
- Duct tape
- Latex gloves
- Tarp
- A means to attach the string/cord to the payload items. You may wish to build a payload harness with nylon or polyester webbing/straps, or perhaps attach string to the corners of the payload and tie down with duct tape. Securing metal swivels or rings to the top and bottom of the box (or harness) allows for easy attachment of the payload to the payload train. You might have to experiment a little bit to find the best method of attachment for your specific payload box.
- Space to set up, fill, and release balloon, preferably outdoors on a calm morning
NOTE: For the live tracking device, there are multiple approaches one could use. One approach is to simply purchase a device that provides real-time tracking and data transmission via a satellite network, like the Iridium network. This can be rather expensive ($200–500) and often requires a monthly or annual satellite subscription. There are also companies that sell high-quality, ready-to-go tracking devices specifically for ballooning (e.g., https://stratostar.com/), although these can be much more expensive. A cheaper option could be the $300 flight computer from High Altitude Science (https://www.highaltitudescience.com/products/eagle-flight-computer). Many of these purchases are one-time purchases to get started and equipment can be reused for future launches.
Teachers who are more tech-savvy may wish to construct their own devices using an Arduino or Raspberry Pi and track the balloon via Ham radio (see https://www.overlookhorizon.com/how-to-launch-weather-balloons/tracking-systems/ or https://www.sparkfun.com/tutorials/185). This can be a significantly cheaper option (<$100), but of course requires some knowledge of electronics, circuits, and Ham radios. The simplest approach is to just track the payload with a SPOT GPS transmitter (see above). However, these types of GPS transmitters update less frequently (every ~5 minutes), often do not operate above ~18,300 m (60,000 ft) above sea level, and do not generally include altitude data. In testing these balloon launches, this curriculum used equipment previously purchased using grant funds from a company called StratoStar. Helium tanks are an additional expense. For teachers in the state of Wyoming, launches and helium are provided by Wyoming NASA Space Grant free of charge and teachers can apply for grants to purchase additional supplies and equipment through our office as well if needed. Teachers in other states may be able to partner with the Space Grant office in their state, with universities engaged in launching weather balloons, or apply for grants to get started in ballooning.
Additional resources for purchasing ballooning equipment:
Worksheets and Attachments
Visit [www.teachengineering.org/activities/view/uow-2546-high-altitude-balloon-launch-cosmic-radiation-activity2] to print or download.Pre-Req Knowledge
- Knowledge of radiation and types of radiation (learned in the associated lesson, Shielding from Cosmic Radiation: Space Agency Scenario).
- Familiarity with their assigned role in their space agency.
- Understand how radiation is measured.
- Identify and describe the types of materials can be used to block cosmic rays.
- Understand high-altitude ballooning and the conditions that the balloon will be exposed to (temperature, pressure, humidity changes, etc.).
- Understand how to build a payload/prototype of a device that attempts to shield radiation at high altitudes.
Introduction/Motivation
By now your teams have designed and built a radiation shield for astronauts at high-altitude. Next it is time to test your prototype by sending it into near space attached to a high-altitude balloon. The short explanation of what we are about to do is in three steps: First, we will put the finishing touches on your payload, prepare for the launch, and invite others to watch the launch. Second, after the balloon has been released, we will track the balloon during its flight. Third, after the balloon bursts and its payloads fall back to Earth, we will retrieve the parachute and payloads.
Putting finishing touches on the payload means that our Engineer(s) and Computer Scientist(s) must verify that the payload is properly secured, that it is powered up properly, and that the sensors and microcomputer are running properly. Is this go, or no-go?
Inviting others to watch the balloon means not only asking the public and local media to attend the actual launch on the ground but might also mean offering the public a view of this mission on our social media accounts. Having a well-informed and interested public is very important to our mission.
Tracking the balloon and recovering the payload require that we have gone through our launch checklists carefully. Have we filled the balloon with the correct volume of gas? If so we should reach our target altitude. Have we run flight scenarios using flight prediction software? Then we should land at a reasonable place to recover our payload. This mission will acquire valuable data for our space program and nation.
Procedure
Background
Each group finalizes its payload for data collection. The balloon is filled with helium. Students and teachers attach payloads, parachute, and tracking device to balloon, launch the balloon, track its path and landing site, then travel to the landing site and retrieve the payloads and parachute. A good step-by-step balloon launch guide can be found at: https://www.overlookhorizon.com/how-to-launch-weather-balloons/. You can view videos of past Wyoming NASA Space Grant launches on our YouTube channel: WYSpaceGrant, see data from past launches, and get additional resources from our website (https://www.youtube.com/channel/UC5zD0W122DzNc0jPhSDL5_Q; http://wyomingspacegrant.org/balloonprogram/). StratoStar.com has additional resources for teachers interested in high-altitude ballooning. Their website is: https://stratostar.com/.
Continue to follow the Payload Manual Guide during this process. The guide provides critical instructions on the payload. See the links in the Multimedia Support and Technology Integration section below for resources on balloon launch, recovery, and more.
Before the Activity
- Select a location outdoors where the balloon may be inflated and released. A large open field, such as a school playground or sports field is generally large enough.
- Run flight prediction software to assist in selecting a day and time for launch with optimal weather conditions. Optimal conditions are clear skies and calm winds (<10 mph). Software is available from https://stratostar.com/
- Notify the school and local media about the launch. Launches are very safe and students typically enjoy watching and counting down the release.
- Ensure the tracking device works and that it can continue to work at low temperatures before release (high altitude).
- Devise a system of attaching the tracking device, parachute, and payloads to the balloon using payload line (string/paracord), harnesses, tape, metal rings, etc. Individual payload items should be separated by at least 0.9 to 1.2 meters (~3-4 ft).
- (Every day within 5 days of launch) Monitor the weather forecast to ensure good conditions for launch day.
- (Every day within a week of launch) Monitor the predicted flight trajectory (see link below) to ensure favorable landing site.
With the Students
- Assist students as necessary to secure their payloads within a padded box, such as a Styrofoam box or cooler (see image below for an example)
- Assist students with (1) assembling the payload train by attaching the payload items (including parachute) to each other with the string/paracord, and (2) attaching the top of the payload train to the balloon. For one example of how to do this, see this video from Stratostar: https://www.youtube.com/watch?v=6_06Q_eWta8. Essentially, you want to tie off the neck of the balloon and then firmly attach the string/paracord at the top of the payload train to the neck of the balloon. We recommend attaching the string to the balloon using zip ties, although that is not the only way this can be done.
- Inflate the balloon. (Here’s a helpful guide: https://www.overlookhorizon.com/how-to-launch-weather-balloons/inflate-weather-balloon/).
- Release the balloon, track its ascent, altitude, path, and descent on a lap top.
- After the balloon bursts, locate and retrieve the parachute and payload after they fall back to Earth.
Vocabulary/Definitions
Arduino: Microcontroller using open-source hardware/software that is a tool for controlling electronics.
electromagnetic radiation: ER composes the waves of the electromagnetic field, which includes visible light, x-rays, infrared, and gamma rays. Cosmic radiation includes gamma rays.
Geiger counter: A device used to detect and measure ionizing radiation.
high-altitude: There are different definitions, but this generally includes 8,000 feet above sea level+. High-altitude balloons can reach altitudes over 100,000 feet.
particle radiation: Particle radiation is made up of alpha and beta particles. An alpha particle is basically a highly ionized helium atom. A beta particle is just a free electron. Alpha is deadly but is stopped much more easily than beta. We are working with cosmic radiation, which is composed primarily of beta.
payload: The container and device(s) used to collect data; necessary to retrieve for complete data analysis, although some data is collected real-time.
prototype: The initial draft of a project and the first step to further improve the project after testing.
Assessment
Pre-Activity Assessment
Quick Recap of Terms: Verbally ask students terms and concepts related to radiation. Confirm that students know the expectations of their roles. The Director should have presented on their team’s (country’s) progress by now.
Activity Embedded (Formative) Assessment
Payload Status: Confirm with each group their payload is ready to launch. Verify that they have tested it on the ground.
Post-Activity (Summative) Assessment
None. If students are cleared for travel, they may all travel and hike to the retrieval site. (Note: the authors were not able to obtain permission for students to cross state lines, so the teacher and a partner teacher secured a substitute teacher for the day and went together to retrieve the payloads.
Investigating Questions
- How does your team’s radiation shield function at high altitude?
- Does the Geiger counter measure higher or lower radiation at higher altitudes?
- Do the radiation measurements increase or decrease at a constant rate?
- How well does the shield reduce radiation counts?
- How do your shielded Geiger counter measurements compare to the control?
Safety Issues
- Students shouldn’t stand too close to the balloon as it is being inflated, especially on windy days. The balloon could start to wobble and hit someone standing too close. About ten feet away is generally a safe distance.
- If students need to stabilize the balloon by holding it gently, make sure they wear gloves so that skin oils do not come in contact with the latex of the balloon.
- Be sure to review FAA regulations for free unmanned balloons: https://www.overlookhorizon.com/flight-safety/.
Troubleshooting Tips
See the Payload Manual Guide for troubleshooting tips regarding the cosmic radiation payload. There is the risk that the student’s Arduino devices malfunction and do not collect any radiation data or that the payload is unrecoverable. If that happens, the follow-up discussion could turn to analyzing the experiment, what may have gone wrong, and what could be improved. Engineers must cope with “failed” experiments and ideally learn from them.
Activity Extensions
Students can track the balloon’s path using GPS and the tracking system. Students can research regulations pertaining to the payload landing on private vs. public land, as well as confirm their payload meets FAA regulations.
Activity Scaling
- For lower grades, teacher may have to track while students look at a shared computer and projector.
- For higher grades, students may track the balloon on their own personal devices, and prepare to hike up to several miles to recover the payload.
Additional Multimedia Support
- Wyoming NASA Space Grant ballooning program
- Wyoming NASA Space Grant ballooning videos
- Overlook Horizon High-Altitude Ballooning (How To Launch Balloons)
- High Altitude Science Ballooning Tutorials
- Stratostar (High-Altitude Balloon Education Company)
- Cambridge University Spaceflight Landing Predictor (flight prediction)
Subscribe
Get the inside scoop on all things TeachEngineering such as new site features, curriculum updates, video releases, and more by signing up for our newsletter!More Curriculum Like This

Through role playing and problem solving, this lesson sets the stage for a friendly competition between groups to design and build a shielding device to protect humans traveling in space. The instructor asks students—how might we design radiation shielding for space travel?

Students analyze radiation data collected from a balloon launched into near space to see if their designed cosmic radiation shielding worked. Students should explain their findings, which might require some research to show if this observation is correct and why. This includes a comparison of the s...

Students design and build a payload that measures the effect of shielding from cosmic radiation. Each payload includes a Geiger counter, data collection device (Arduino and microSD memory card), and uses materials that will shield their Geiger counter from cosmic radiation.

Students analyze cellphone signal data from a high-altitude balloon launch, testing how the strength of the cell signal changes with altitude. Students begin by looking at graphs and discussing the various components, as well as different types of graphs. Students then can practice collecting, plott...
Copyright
© 2021 by Regents of the University of Colorado; original © 2019 University of WyomingContributors
Jeff Bell; Mary Block; Garrett Burrows; Phil Bergmaier; Trina Kilty; Andrea Burrows; Kevin Kilty; Kate Muir-Welsh; Shawna McBride; Jim StithSupporting Program
Wyoming NASA Space Grant Consortium, University of WyomingAcknowledgements
Much of the equipment and training to develop the Wyoming NASA Space Grant balloon program were provided by StratoStar.
This material was developed based upon work supported by the National Science Foundation under grant no. 1821566—the LIFT (Learning to Integrate Fundamentals through Teaching) Project, Wyoming NASA Space Grant Consortium, University of Wyoming. Any opinions, findings and conclusions or recommendations expressed in this material are those of the authors and do not necessarily reflect the views of the National Science Foundation.
Last modified: March 29, 2022
User Comments & Tips