Quick Look
Grade Level: 9 (9-12)
Time Required: 3 hours
(can be split into different sessions)
Expendable Cost/Group: US $20.00
Group Size: 2
Activity Dependency: None
Subject Areas: Measurement, Physical Science, Physics, Problem Solving, Science and Technology
NGSS Performance Expectations:
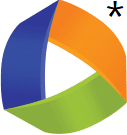
HS-ETS1-2 |
Summary
Students create and analyze composite materials with the intent of using the materials to construct a structure with optimal strength and minimal density. The composite materials are made of puffed rice cereal, marshmallows and chocolate chips. Teams vary the concentrations of the three components to create their composite materials. They determine the material density and test its compressive strength by placing weights on it and measuring how much the material compresses. Students graph stress vs. strain and determine Young's modulus to analyze the strength of their materials.Engineering Connection
Students play the role of composite material engineers as they create the best (lightest, yet sturdiest) composite material to be used in an area that suffers from severe ground subsidence, such as New Orleans.
Material engineers choose constituents (that is, ingredients) for composites based on their density and rigidity. Engineers design new materials and perform compression tests on them to determine their strength. Engineers analyze stress vs. strain graphs to find Young's modulus, thereby determining the material stiffness. Based on the material's strength and the extent of its elastic region, composite engineers determine what materials would be optimal for a given application.
Learning Objectives
After this activity, students should be able to:
- Design a strong, yet lightweight, composite material.
- Construct and analyze a stress vs. strain graph for a composite material.
- Compare different composite materials and explain which is the most desirable building material, considering strength and density.
Educational Standards
Each TeachEngineering lesson or activity is correlated to one or more K-12 science,
technology, engineering or math (STEM) educational standards.
All 100,000+ K-12 STEM standards covered in TeachEngineering are collected, maintained and packaged by the Achievement Standards Network (ASN),
a project of D2L (www.achievementstandards.org).
In the ASN, standards are hierarchically structured: first by source; e.g., by state; within source by type; e.g., science or mathematics;
within type by subtype, then by grade, etc.
Each TeachEngineering lesson or activity is correlated to one or more K-12 science, technology, engineering or math (STEM) educational standards.
All 100,000+ K-12 STEM standards covered in TeachEngineering are collected, maintained and packaged by the Achievement Standards Network (ASN), a project of D2L (www.achievementstandards.org).
In the ASN, standards are hierarchically structured: first by source; e.g., by state; within source by type; e.g., science or mathematics; within type by subtype, then by grade, etc.
NGSS: Next Generation Science Standards - Science
NGSS Performance Expectation | ||
---|---|---|
HS-ETS1-2. Design a solution to a complex real-world problem by breaking it down into smaller, more manageable problems that can be solved through engineering. (Grades 9 - 12) Do you agree with this alignment? |
||
Click to view other curriculum aligned to this Performance Expectation | ||
This activity focuses on the following Three Dimensional Learning aspects of NGSS: | ||
Science & Engineering Practices | Disciplinary Core Ideas | Crosscutting Concepts |
Design a solution to a complex real-world problem, based on scientific knowledge, student-generated sources of evidence, prioritized criteria, and tradeoff considerations. Alignment agreement: | Criteria may need to be broken down into simpler ones that can be approached systematically, and decisions about the priority of certain criteria over others (trade-offs) may be needed. Alignment agreement: |
Common Core State Standards - Math
-
Interpret expressions that represent a quantity in terms of its context
(Grades
9 -
12)
More Details
Do you agree with this alignment?
-
Use units as a way to understand problems and to guide the solution of multi-step problems; choose and interpret units consistently in formulas; choose and interpret the scale and the origin in graphs and data displays.
(Grades
9 -
12)
More Details
Do you agree with this alignment?
-
Define appropriate quantities for the purpose of descriptive modeling.
(Grades
9 -
12)
More Details
Do you agree with this alignment?
-
Choose a level of accuracy appropriate to limitations on measurement when reporting quantities.
(Grades
9 -
12)
More Details
Do you agree with this alignment?
-
Solve quadratic equations in one variable.
(Grades
9 -
12)
More Details
Do you agree with this alignment?
-
Solve linear equations and inequalities in one variable, including equations with coefficients represented by letters.
(Grades
9 -
12)
More Details
Do you agree with this alignment?
-
Summarize, represent, and interpret data on a single count or measurement variable
(Grades
9 -
12)
More Details
Do you agree with this alignment?
International Technology and Engineering Educators Association - Technology
-
Illustrate that there are multiple approaches to design.
(Grades
3 -
5)
More Details
Do you agree with this alignment?
-
Technological problems must be researched before they can be solved.
(Grades
9 -
12)
More Details
Do you agree with this alignment?
-
Conduct research to inform intentional inventions and innovations that address specific needs and wants.
(Grades
9 -
12)
More Details
Do you agree with this alignment?
State Standards
New York - Science
-
Design a solution to a complex real-world problem by breaking it down into smaller, more manageable problems that can be solved through engineering.
(Grades
9 -
12)
More Details
Do you agree with this alignment?
Materials List
Each group needs:
- 4-5 jumbo marshmallows
- ½ stick butter
- 4 cups Rice Krispies® (or similar puffed rice cereal)
- 4-5 small cupcake wrappers (aka paper "cupcake cups")
- 2 cups chocolate chips
- 2 zinc-plated nails
- 1 muffin pan (disposable pans suffice)
- 1 plastic spoon
- 1 ruler
- 1 wooden block, ~3 inches x 3 inches x ½ inch (8 cm x 8 cm x 1 cm)
- 1 microwave-safe container
- computer with Microsoft Excel® or similar software
For the teacher demonstration:
- 1 jumbo marshmallow
- 1 ruler
- 1 wooden block, ~3 inches x 3 inches x ½ inch (8 cm x 8 cm x 1 cm)
- Rebuilding New Orleans Composite Materials Lab Handout, one per student
To share with the entire class:
- microwave
- balance/scale
- (optional) flexi force sensor; see https://learn.adafruit.com/force-sensitive-resistor-fsr/using-an-fsr
- (optional) microcontroller, such as the Arduino Uno R3 for $25 at https://www.adafruit.com/product/50
- 1 weight set with 4-5 different weights 50 g – 500 g
Worksheets and Attachments
Visit [www.teachengineering.org/activities/view/nyu_neworleans_activity1] to print or download.Pre-Req Knowledge
Students should be able to:
- Graph data.
- Draw a best-fit line or add a best-fit line to a computer-generated graph.
- Determine and analyze the equation of a line from a graph.
- Find and calculate the density of an object.
Introduction/Motivation
Good morning, everyone! Who can tell me some things about the city of New Orleans? (Write students' answers on the classroom board; possible answers: huge Mardi Gras celebration, Brad Pitt and Angelina Jolie live there, Cajun cuisine, creole culture, etc.). Okay, now tell me some more environmental facts. (Possible answers: It is below sea level, it is shrinking, Hurricane Katrina hit there, the levees broke.) Excellent! It is a true fact that the elevation of New Orleans is below sea level. Who can tell me exactly what that means? (Possible answers: The sea is higher than the land, the land is lower than the sea water, etc.) It seems confusing and impossible. Let's watch a short video that shows what this concept means and explains how we were able to build an entire city below sea level. (Play this five-minute video: http://www.pbslearningmedia.org/resource/ess05.sci.ess.watcyc.hurricane/hurricanes-new-tools-for-predicting/.) As you saw, this video was filmed before Hurricane Katrina, but we know now that the devastating fears described by the city's engineers and other officials actually occurred during and after Hurricane Katrina. We also know that people returned to the water-devastated city of New Orleans and have started to rebuild their homes, businesses, and scenic and recreational areas. Many controversial opinions exist about the cost attributed with rebuilding a city that is and continues to be at such risk. (Play this two-minute video: http://www.cbsnews.com/news/new-orleans-is-sinking/.).
So, we know that people have decided to return to their homes (or new homes), and the city has attempted to return to as normal an existence as is possible. Your role, as composite material engineers, is to create a new building material that may help save the people of New Orleans and government funds should another natural disaster occur in the future.
Today, as a materials engineer, you have been asked by the Army Corps of Engineers to develop this innovative material because even once new, higher levees are built, the ground that New Orleans sits on is still very swampy. This means that subsidence occurs. Subsidence is the gradual caving in or sinking of an area of land. In New Orleans, a lot of subsidence occurs each year. Using dense materials, such as steel and bricks, actually speeds up the rate of subsidence, and another deterrent method, undergirding—that is, securing the land from the underside—is cost prohibitive due to the large size of the swampy land mass.
To complete this task, begin by creating three different composite materials made of a variety of materials. The reason engineers create composites is because they (composites) minimize the weaknesses of individual materials while maximizing their strengths. For example, concrete is a mixture of cement, rocks, sand and water—a composite of these four materials. Rocks alone are very strong, but are not cohesive; cement is weaker and less dense than rocks. When we combine all the materials at very specific quantities, we get a new material, called a composite, which is strong and suitable for building. And, if we find ways use more inexpensive and/or natural materials, the composite is even better.
For this lab, first create samples in which you vary the amount of the individual materials based on what you think will create the lightest, sturdiest composite. Next, measure each sample's mass and calculate its volume to determine its density. Finally, determine the strength of your sample by graphing stress (force per unit area = pressure) vs. strain (amount the sample compressed/original length of sample). Basically, we want to find out how strong each sample is by applying force to deform it. The more force required to compress the material, the stronger the material.
Teacher Demonstration
To start off, I am going to demo the procedures for this activity with—a jumbo marshmallow! (The procedures are described in detail in the Young's Modulus of a Marshmallow Lab Procedures. Follow the full procedure and engage students when possible by having them volunteer to help with the various steps of the procedure. Once the demonstration is complete, students should understand how they will conduct the lab.)
Procedure
Background
Young's modulus is the ratio of a material's stress to strain. It is a measure of a material's stiffness. A material with a large Young's modulus does not stretch or compress a lot when pressure is applied to it. Engineers analyze and compare materials using Young's modulus because this stress-to-strain ratio is dependent on the material's properties themselves, not the physical size of the material. This is an important factor for engineers to know in determining which materials to use in fabricating buildings, cars, bridges, computers, roads, etc.
A composite material is one that is made by combining at least two materials that have different properties. Engineers design composites to create materials that are stronger, lighter or less expensive. One method of designing a strong and lightweight composite material is to create syntactic foam, in which hollow particles are added to matrix material. The matrix is a material that binds the particles together. The matrix is often a polymer, but can also be metal or ceramic. The hollow particles are called microballoons. In this lab, marshmallows serve as the matrix (or binder), and the puffed rice cereal serves as the microballoons (hollow particles).
Example composite materials:
- Wood – combination of cellulose fibers (a polymer) in a matrix of lignin. Cellulose is found in cotton also, but it does not create a strong material without lignin.
- Bone – combination of mostly calcium phosphate in a matrix of collagen (a protein). Collagen is also found in hair and fingernails, but is not very strong on its own.
- Concrete – combination of water, aggregate (sand, crushed stone, gravel or shale) in a matrix of cement (mixture of clay and limestone that is used to bind the materials together).
- Plywood – sheets of wooden fibers in a matrix of glue. The sheets of wooden fibers are layered in a crisscrossed way to improve stability.
- Papier-mâché – small pieces of paper in a matrix of glue.
- Fiberglass – woven glass threads in a matrix of plastic.
Products made using syntactic foams:
- Soccer ball created for the 2014 World Cup. Link with article and video: https://www.livescience.com/46299-microscopic-analysis-brazuca-world-cup-ball.html
- Automotive metal foam – Fly ash produced by coal power plants contains hollow spheres that can be mixed with metal to create metal foam that is lighter and stronger.
- Submarines – Syntactic foams are buoyant, have low moisture absorption and high compressive strength. The submarine Alvin HOV, that located the Titanic, was made using syntactic foams.
- Aircraft – Boeing and Airbus Americas use syntactic foams to strengthen hollow areas.
- Fire protective roof – XFlam produces flame-resistant materials that are thermally insulating.
Subsidence is the sinking of the ground. Subsidence can be sudden, as in the case of a sinkhole, or occur slowly over time.
Subsidence can occur as a result of:
- Carbon dioxide becoming dissolved in water, forming carbonic acid. When it rains, the carbonic acid dissolves calcite in limestone. Caves form near the water table as a result of this process. If the water table lowers, then the cave roof can fall in, forming a sinkhole. The water table can lower due to water being pumped up to the surface.
- Salt or coal mining that removes material from beneath the surface, making the ground weak. This "weakness" can cause the surface to sink.
- The removal of water, natural gas or oil from the ground, which causes empty spaces into which sediment can settle.
- Cities being built in delta areas (where rivers enter the ocean), near rivers that flood, in coastal marshlands, or on unconsolidated sediments. The added weight due to the construction of buildings and roads compacts the sediment.
The following article is a detailed explanation of the causes of subsidence and lists cities around the world that are facing this issue and the cause of it: http://www.tulane.edu/~sanelson/Natural_Disasters/subsidence.htm
Analyzing the Strength of a Material
To analyze the strength of a material, engineers apply a force to the material and then measure how much the material stretches or compresses. Rather than graphing force vs. distance stretched (known as Hooke's law), engineers prefer to graph stress vs. strain. Stress is the force per unit area (measured in Pascals), and strain (dimensionless) is the ratio of the change in length to the original length. Graphing stress vs. strain has the advantage of being material specific and does not change as a result of material thickness. The slope of a stress vs. strain graph is called Young's modulus or the modulus of elasticity. The larger a material's Young's modulus, the more rigid the material. If material A has a larger Young's modulus than material B, it means that material A stretches less (it is more rigid) than material B for the same amount of pressure applied. Thus, knowing a material's Young's modulus tells us about the material's strength.
Typically, stress is directly proportional to strain until a certain amount of pressure is applied. The range over which stress and strain are directly proportional is when the material is behaving elastically. At that time, the material returns to its initial size and shape if the force applied is removed. Imagine a spring or rubber band going back to its original shape after small forces are removed. However, if too much force is applied to a spring, it gets 'bent out of shape,' and never returns to its original size. Then, the material is said to be in its plastic state, and the relationship between stress and strain is no longer linear. If a material breaks before it leaves its elastic state (and there was no indication that it would break before it did), we call the material brittle. If the material breaks after being in its plastic state, then we call it ductile.
Before the Activity
- Gather materials and make copies of the Rebuilding New Orleans Composite Materials Lab Handout.
- Set up a marshmallow squashing station (Figure 1) for demo purposes, which can also serve as a model for students to set up their own testing stations.
Figure 1. The demonstration setup: a marshmallow squashing station.
With the Students
- Provide students with the lab handout and have them read through it.
- In pairs, have students determine the amount of marshmallows, butter, puffed rice cereal and chocolate chips to add to make each of their three composite materials.
- Direct them to complete steps 1-9 under Part 1 – Design of the lab handout.
- Have students continue to Part 2 – Analyze the Material of the lab handout, completing steps 1-6 by performing the necessary measurements and calculations and completing the data table.
- Then have students complete Part 3 – Strength Test to determine the stress and strain of each of the three composite materials as different masses are applied.
- Using Microsoft Excel® or similar software, students graph the stress vs. strain for each composite material on the same set of axes, including a line of best fit and an equation of the line for each of the three composite materials' data.
- Using the slope of each line, students determine Young's modulus for each of the three materials and then answer the Discussion and Extension Questions on the lab handout.
- On the classroom board, make a blank chart and have each team write in itssamples' density and modulus.
Post-Lab Wrap-Up
Now that each group has tested their materials, let's compare and discuss our class results. Which composite is the best candidate to use as a new building material for apartments, hospitals, schools and other buildings in New Orleans? (Students select from the class chart that shows each sample's density and modulus.) Obviously, we have used edible materials that could not really be used as building materials. What are some real-world materials or possible composites that you think could work? (Possible answers: Metal matrix composite, such as carbon fiber in an aluminum matrix or fiber-reinforced polymers.)
Note: At the conclusion of the class discussion, instruct students to complete the "L" of the Rebuilding New Orleans KWL Worksheet.
Vocabulary/Definitions
composite material: A material composed of two or more materials. The properties of the composite are different from those of the components that make it up. The components of the composite do not dissolve in each other and are distinguishable from each other.
compression: A force that pushes inward on a material.
elastic: A material's ability to return to its original shape and length after being stretched or compressed.
matrix: Material in a composite that is used to bind the materials together.
strain : The ratio of a material's stretched or compressed length to its original length (deformation due to stress).
stress: The pressure (force per unit area) acting to pull apart or compress a material.
subsidence: The gradual caving in or sinking of an area of land.
syntactic foam: Type of composite material composed of hollow spheres inside a matrix.
Young's modulus : (modulus of elasticity) Ratio of stress to strain; a measure of a material's stiffness. For many materials, the strain is directly proportional to the stress applied. When the stress applied is within this linear region, the material is behaving elastically. That is, that when the stress is removed, the material returns to its original shape and size.
Assessment
Pre-Lesson Assessment
Rebuilding New Orleans KWL: Have students complete the K and W portions of the Rebuilding New Orleans KWL Worksheet before presenting the Motivation/Introduction content. Have students complete L portsion after the conclusion of the lab and the class discussion.
Activity Embedded Assessment
Rebuilding New Orleans Composite Materials Lab: Students complete the Rebuilding New Orleans Composite Materials Lab Handout to find the density of their samples as well as create stress vs. strain graphs of their composites. Students collect and analyze data in order to determine which of their composites is the strongest, yet lightest. Review their completed handouts (data, calculations, answers to the questions) to gauge their engagement and depth of comprehension.
Post-Activity Assessment
Vocabulary Homework: Assign students to either create a vocabulary foldable or set of flash cards in which they define the vocabulary words as well as show their understanding by creating a visual or sentence for each term.
Class Discussion: As a class, have students share group results so they can discuss and determine which composite would be the best and worst to use in New Orleans.
Stress vs. Strain Analysis: Assign students to analyze stress vs. strain graphs, guided by the Stress vs. Strain Worksheet, for aggregate, concrete and cement in order apply the concepts learned in the lab to real-world construction materials.
Activity Extensions
Cost Efficiency Comparison: Have students use the Unit Price List to determine which of their composites was the most cost-efficient.
Activity Scaling
For lower grades, do the activity as is, but apply a single force to each sample and compare how much each sample compresses. Students could also find the ratio of the mass applied to the distance it compresses.
Subscribe
Get the inside scoop on all things TeachEngineering such as new site features, curriculum updates, video releases, and more by signing up for our newsletter!References
Composite Materials. Advancing the Chemical Sciences, Royal Society of Chemistry. Accessed July 28, 2014. (Source of background information and some vocabulary definitions) http://www.rsc.org/Education/Teachers/Resources/Inspirational/resources/4.3.1.pdf
Dictionary.com. IAC. Accessed July 24, 2014. (Source of some vocabulary definitions, with some adaptation) http://www.dictionary.com
Engineering Toolbox. Accessed July 28, 2014. (Source of some vocabulary definitions with some adaptation) http://www.engineeringtoolbox.com/stress-strain-d_950.html
Gou, Jan. Advanced Materials Science, University of South Alabama. Accessed July 24, 2014. (Source of background information) http://www.southalabama.edu/engineering/mechanical/faculty/gou/Teaching/ME582/ME%20582%20F06%20-%20Chapter%201%20Introduction.pdf
Gupta, Nikhil. Zeltmann, Steven. Shunmugasamy, Vasanth Chakravarthy. Pinisetty, Dinesh. "Application of Polymer Matrix Syntactic Foams." The Journal of the Minerals, Metals, and Materials Society. 2 November 2013.
Nelson, Stephen A. Subsidence: Dissolution & Human Related Causes. Updates July 9, 2012. Tulane University. Accessed August 4, 2014. (Source of background information on subsidence) http://www.tulane.edu/~sanelson/Natural_Disasters/subsidence.htm
New York State Board of Regents. Physical Setting/ Earth Science Core Curriculum. The University of the State of New York, State Education Department. Accessed July 31, 2014. (Source of information about state standards). http://www.p12.nysed.gov/ciai/mst/pub/phycoresci.pdf
New York State Board of Regents. Physical Setting/ Physics Core Curriculum. The University of the State of New York, State Education Department. Accessed July 31, 2014. (Source of information about state standards). http://www.p12.nysed.gov/ciai/mst/pub/phycoresci.pdf
Pestka II, Kenneth A. "Young's Modulus of a Marshmallow." The Physics Teacher. February 2008: Volume 46, p. 140.
Copyright
© 2015 by Regents of the University of Colorado; original © 2014 Polytechnic Institute of New York UniversityContributors
Charisse Nelson, Sarah WigodskySupporting Program
SMARTER RET Program, Polytechnic Institute of New York UniversityAcknowledgements
This activity was developed by the Science and Mechatronics Aided Research for Teachers with an Entrepreneurial ExpeRience (SMARTER): A Research Experience for Teachers (RET) Program in the School of Engineering funded by National Science Foundation RET grant no. 1132482. However, these contents do not necessarily represent the policies of the NSF, and you should not assume endorsement by the federal government.
Last modified: February 12, 2020
User Comments & Tips