Quick Look
Grade Level: 10 (9-11)
Time Required: 1 hours 45 minutes
(Conduct 1, 2 or 3 challenges; each takes 75-100 mins or 1.5-2 class periods)
Expendable Cost/Group: US $0.16
Group Size: 2
Activity Dependency: None
Subject Areas: Biology, Life Science, Measurement
NGSS Performance Expectations:
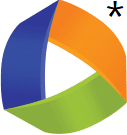
HS-ETS1-2 |
HS-ETS1-3 |
HS-LS1-1 |
HS-LS1-2 |
Summary
Students act as if they are biological engineers following the steps of the engineering design process to design and create protein models to replace the defective proteins in a child’s body. Jumping off from a basic understanding of DNA and its transcription and translation processes, students learn about the many different proteins types and what happens if protein mutations occur. Then they focus on structural, transport and defense proteins during three challenges posed by the R&D bio-engineering hypothetical scenario. Using common classroom supplies such as paper, tape and craft sticks, student pairs design, sketch, build, test and improve their own protein models to meet specific functional requirements: to strengthen bones (collagen), to capture oxygen molecules (hemoglobin) and to capture bacteria (antibody). By designing and testing physical models to accomplish certain functional requirements, students come to understand the relationship between protein structure and function. They graph and analyze the class data, then share and compare results across all teams to determine which models were the most successful. Includes a quiz, three worksheets and a reference sheet.Engineering Connection
Biological engineers design solutions in the form of machines, structures, processes and instrumentation that address problems involving living biological systems including plants, animals and microbes. In this activity, like bio-engineers, students imagine and create structures that model the functions of specific proteins and then test how well they perform, all while guided by the engineering design process.
Learning Objectives
After this activity, students should be able to:
- Explain that proteins are composed of amino acids.
- Describe the functions of three proteins.
- Brainstorm, sketch and build model proteins using common materials.
- After testing, modify their models to improve effectiveness and better meet requirements.
- Keep records of the design and proficiency of the tested models.
- Communicate to others the model design and its strengths and weaknesses.
- Compare the strengths and weaknesses of other models to their own and reflect on ideas that might improve their own designs.
Educational Standards
Each TeachEngineering lesson or activity is correlated to one or more K-12 science,
technology, engineering or math (STEM) educational standards.
All 100,000+ K-12 STEM standards covered in TeachEngineering are collected, maintained and packaged by the Achievement Standards Network (ASN),
a project of D2L (www.achievementstandards.org).
In the ASN, standards are hierarchically structured: first by source; e.g., by state; within source by type; e.g., science or mathematics;
within type by subtype, then by grade, etc.
Each TeachEngineering lesson or activity is correlated to one or more K-12 science, technology, engineering or math (STEM) educational standards.
All 100,000+ K-12 STEM standards covered in TeachEngineering are collected, maintained and packaged by the Achievement Standards Network (ASN), a project of D2L (www.achievementstandards.org).
In the ASN, standards are hierarchically structured: first by source; e.g., by state; within source by type; e.g., science or mathematics; within type by subtype, then by grade, etc.
NGSS: Next Generation Science Standards - Science
NGSS Performance Expectation | ||
---|---|---|
HS-ETS1-2. Design a solution to a complex real-world problem by breaking it down into smaller, more manageable problems that can be solved through engineering. (Grades 9 - 12) Do you agree with this alignment? |
||
Click to view other curriculum aligned to this Performance Expectation | ||
This activity focuses on the following Three Dimensional Learning aspects of NGSS: | ||
Science & Engineering Practices | Disciplinary Core Ideas | Crosscutting Concepts |
Design a solution to a complex real-world problem, based on scientific knowledge, student-generated sources of evidence, prioritized criteria, and tradeoff considerations. Alignment agreement: | Criteria may need to be broken down into simpler ones that can be approached systematically, and decisions about the priority of certain criteria over others (trade-offs) may be needed. Alignment agreement: |
NGSS Performance Expectation | ||
---|---|---|
HS-ETS1-3. Evaluate a solution to a complex real-world problem based on prioritized criteria and trade-offs that account for a range of constraints, including cost, safety, reliability, and aesthetics, as well as possible social, cultural, and environmental impacts. (Grades 9 - 12) Do you agree with this alignment? |
||
Click to view other curriculum aligned to this Performance Expectation | ||
This activity focuses on the following Three Dimensional Learning aspects of NGSS: | ||
Science & Engineering Practices | Disciplinary Core Ideas | Crosscutting Concepts |
Evaluate a solution to a complex real-world problem, based on scientific knowledge, student-generated sources of evidence, prioritized criteria, and tradeoff considerations. Alignment agreement: | When evaluating solutions it is important to take into account a range of constraints including cost, safety, reliability and aesthetics and to consider social, cultural and environmental impacts. Alignment agreement: | New technologies can have deep impacts on society and the environment, including some that were not anticipated. Analysis of costs and benefits is a critical aspect of decisions about technology. Alignment agreement: |
NGSS Performance Expectation | ||
---|---|---|
HS-LS1-1. Construct an explanation based on evidence for how the structure of DNA determines the structure of proteins which carry out the essential functions of life through systems of specialized cells. (Grades 9 - 12) Do you agree with this alignment? |
||
Click to view other curriculum aligned to this Performance Expectation | ||
This activity focuses on the following Three Dimensional Learning aspects of NGSS: | ||
Science & Engineering Practices | Disciplinary Core Ideas | Crosscutting Concepts |
Construct an explanation based on valid and reliable evidence obtained from a variety of sources (including students' own investigations, models, theories, simulations, peer review) and the assumption that theories and laws that describe the natural world operate today as they did in the past and will continue to do so in the future. Alignment agreement: | Systems of specialized cells within organisms help them perform the essential functions of life. Alignment agreement: All cells contain genetic information in the form of DNA molecules. Genes are regions in the DNA that contain the instructions that code for the formation of proteins, which carry out most of the work of cells.Alignment agreement: | Investigating or designing new systems or structures requires a detailed examination of the properties of different materials, the structures of different components, and connections of components to reveal its function and/or solve a problem. Alignment agreement: |
NGSS Performance Expectation | ||
---|---|---|
HS-LS1-2. Develop and use a model to illustrate the hierarchical organization of interacting systems that provide specific functions within multicellular organisms. (Grades 9 - 12) Do you agree with this alignment? |
||
Click to view other curriculum aligned to this Performance Expectation | ||
This activity focuses on the following Three Dimensional Learning aspects of NGSS: | ||
Science & Engineering Practices | Disciplinary Core Ideas | Crosscutting Concepts |
Develop and use a model based on evidence to illustrate the relationships between systems or between components of a system. Alignment agreement: | Multicellular organisms have a hierarchical structural organization, in which any one system is made up of numerous parts and is itself a component of the next level. Alignment agreement: | Models (e.g., physical, mathematical, computer models) can be used to simulate systems and interactions—including energy, matter, and information flows—within and between systems at different scales. Alignment agreement: |
Common Core State Standards - Math
-
Reason abstractly and quantitatively.
(Grades
K -
12)
More Details
Do you agree with this alignment?
-
Summarize, represent, and interpret data on a single count or measurement variable
(Grades
9 -
12)
More Details
Do you agree with this alignment?
-
Represent data on two quantitative variables on a scatter plot, and describe how the variables are related.
(Grades
9 -
12)
More Details
Do you agree with this alignment?
International Technology and Engineering Educators Association - Technology
-
Students will develop an understanding of the attributes of design.
(Grades
K -
12)
More Details
Do you agree with this alignment?
-
Students will develop an understanding of the role of troubleshooting, research and development, invention and innovation, and experimentation in problem solving.
(Grades
K -
12)
More Details
Do you agree with this alignment?
-
Students will develop abilities to apply the design process.
(Grades
K -
12)
More Details
Do you agree with this alignment?
-
Students will develop an understanding of engineering design.
(Grades
K -
12)
More Details
Do you agree with this alignment?
State Standards
North Dakota - Math
-
Reason abstractly and quantitatively.
(Grades
K -
12)
More Details
Do you agree with this alignment?
-
Represent data on two quantitative variables on a scatter plot, and describe how the variables are related.
(Grades
9 -
12)
More Details
Do you agree with this alignment?
-
Summarize, represent, and interpret data on a single count or measurement variable
(Grades
9 -
12)
More Details
Do you agree with this alignment?
North Dakota - Science
-
Explain how models can be used to illustrate scientific principles
(Grades
9 -
10)
More Details
Do you agree with this alignment?
-
Explain the relationship between protein structure and function
(Grades
9 -
10)
More Details
Do you agree with this alignment?
Materials List
Each student needs:
Each group needs:
- Engineering Design Drawing Rubric
- computer with Internet access, for looking at specific websites to answer worksheet questions; alternatively, conduct the research as a class using a projector
To share with the entire class:
- (optional) capability to show the class an online website about proteins (in addition to or instead of the reference sheet), and other websites if worksheet research is done as a class
Note: Realistically, all three protein models could be made with just paper and masking tape, but the addition of materials like toothpicks, drinking straws and craft sticks gives the models more support and strength. Twine, string and paperclips are useful for connecting parts. So think of the materials list below as a suggestion; feel free to alter it, depending on supplies you may already have in your classroom. For instance, for the structural protein materials list, you could replace the drinking straws with coffee stir sticks, reduce the number or omit paperclips, and/or provide different kinds of paper.
For making structural protein models (challenge 1)
Each group needs:
- 10 sheets of copy paper
- 10 plastic drinking straws
- 10 paperclips, either all small or all large
- a few books of similar weight to use as weights for structure testing, textbooks work great; you may need as much as 200 pounds of books for the final stress testing
- masking tape, 1 roll
- Protein Shapes Affect Their Functions: Structural Worksheet, one per student
- 1 ruler, for measuring height, not part of the model fabrication
- scissors, used only as a tool, not part of the model structure
To share with the entire class (challenge 1):
- scale, for weighing books
For making transport protein models (challenge 2)
Each group needs:
- masking tape, 1 roll
- string, 2 feet (~61 cm)
- 2 paper plates, any size
- construction or brown wrapping paper
- 1 paper lunch bag
- saran/plastic wrap, 2 feet (~61 cm)
- 10 Popsicle/craft sticks or wooden cocktail sticks
- 10 wooden toothpicks, either flat or round style
- Protein Shapes Affect Their Functions: Transport Worksheet, one per student
- 4 mini marshmallows, for testing only, not part of model structure
- scissors, used only as a tool
To share with entire class (challenge 2):
- 1 bag mini marshmallows
- a three-stage testing area composed of an oxygen (mini marshmallow) dispenser, dispensing station (lungs) and dumping station (cells), made in advance by the teacher, such as four cardboard boxes: a smaller one with holes (to hold the marshmallows and shake them out), a bigger “lungs” box underneath it (to catch stray marshmallows), a smaller box inside the big box to serve as an elevated stand, and a fourth “cells” box where students dump their models after counting the number of captured marshmallows; see examples in Figure 4 and the Procedure section for details
- scrap paper, marker and tape, to label two boxes “LUNGS” and “CELLS”
For making defense protein models (challenge 3)
Each group needs:
- masking tape, 1 roll
- twine or string, 1 foot (~30 cm)
- construction or brown wrapping paper
- aluminum foil, 1 foot (~30 cm)
- saran wrap, 1 foot (~30 cm)
- 1 paper bag
- 1 or 2 balloons, small or medium size
- 10 Popsicle sticks
- Protein Shapes Affect Their Functions: Defense Worksheet, one per student
- scissors, used only as a tool
To share with entire class (for challenge 3):
- shredded paper, placed on a 2 x 4-foot area of the floor; such as paper from the recycling bin run through a cross-shredder (not strips)
- scale or triple beam balance, for measuring mass of paper and/or protein models
Worksheets and Attachments
Visit [www.teachengineering.org/activities/view/nds-2224-bio-engineering-proteins-dna-modeling-testing] to print or download.Pre-Req Knowledge
A basic understanding of DNA, traits, mutations, parts of the cell such as ribosomes, and the basic steps of the engineering design process.
Introduction/Motivation
You have learned that DNA controls your traits, but how does it control your traits? (Listen to student answers.) DNA controls your traits through a process called transcription. DNA makes a molecule of RNA called messenger RNA. Messenger RNA then leaves the nucleus and goes to the part of the cell that makes proteins, which is the _______? (Answer: Ribosome) In a process called translation, messenger RNA works together with the ribosome and another molecule called transfer RNA to put amino acids together to form proteins. Ultimately, DNA controls what proteins are made.
What do you know about proteins? What are some examples of proteins? (Write student answers on the classroom board. When students have nothing else to add, hand out the reference sheet and/or bring up the Types of Proteins website at https://learn.genetics.utah.edu/content/basics/proteintypes/. The main web page has buttons for the following protein types: enzymes, and structural, signaling, regulatory, transport, sensory, motor, defense, and storage proteins.) (optional: Have students research protein types and their functions and share what they learn with the rest of the class. Or, assign a protein type for each group to research and share.)
Here are nine basic types of proteins. (Click on a few of the buttons to show students some example proteins.) What do you think would happen to a person if ______ protein was made incorrectly? (Listen to student ideas. Then share some examples.) For example, if a mutation occurred in a structural protein such as collagen, a person might have brittle bones or skin that ripped easily. A mutation in the enzyme lactase would prevent infants from being able to digest the lactose present in milk.) (optional: Have students research to learn the diseases that result from protein mutations.) (Keep up the website projection because students will refer to it again.)
(Next, before starting on the activity challenges, administer the pre-quiz to verify students’ understanding of proteins.)
(Continue by presenting the following hypothetical scenario to the class to introduce the engineering design challenge.) A couple has just learned that they have given birth to a child that has three different diseases. One is a brittle bone disease called type IV osteogenesis imperfecta in which the body makes enough collagen but it is malformed. The second disease, hypoxemia, is when hemoglobin does not bring the correct amount of oxygen to the cells. Finally, because the child has severe combined immunodeficiency (SCID), it must be kept in a sterile environment because its ability to fight disease is very weak.
Working as if you are biological engineers, your design challenge is to “cure” this child by creating proteins to replace the defective proteins in the child’s body. You will be given amino acids—the building materials—to create each prototype protein model needed to keep the child healthy as it grows.
- The first protein needs to be engineered for strength. Using the amino acids, create a structure that can hold books 1.5-2 inches above the ground.
- The second protein needs to hold as many oxygen molecules as possible using the given materials.
- The third protein must capture as many bacteria as possible using the given materials.
You will be making models of healthy protein structures. We will test and compare everyone’s protein models across all teams in the class to see if you have made a healthy protein or a mutated one.
(Next, hand out the structural worksheet have students read the introduction either together or individually. Continue on as described in the Procedure section.)
Procedure
Activity Overview
This hands-on engineering design activity consists of three different challenges—making prototype models of structural, transport and defense proteins. Expect each challenge to take 75-100 minutes (~1.5 – 2 class periods each), depending on how quickly students come up with ideas, how many times they redesign and how many student groups are testing. If you have time for only one challenge, conduct the structural protein challenge because it is set up to be standalone. Very little teacher preparation of materials is required. The transport and defense proteins challenges build on the structural proteins challenge. If you have time for two activities, first do the structural one and then either the transport or defense challenge.
In getting the materials to students for any of these design challenges, keep in mind that if you prepackage each group’s supplies—that is, organize them in bags, boxes or bowls—they may ask to use the packaging itself as part of the construction materials for their designs. So, decide in advance if you will permit this. Be sure to clarify that tools such as rulers and scissors are not permitted to be used as building materials.
The real-world materials for protein engineering cost much more than the basic supplies used for this student activity. To mirror that real-world constraint, students are given set amounts of materials. If a team damages a material and wants to replace it, it must be returned. If they request an item that is not on the list of available materials, it might be possible to return some in-hand materials in exchange for the requested item. Or students might pitch for a “research grant” to obtain additional funds to purchase materials. In the open-ended hypothetical scenario of this activity, it is up to the teacher to determine the limits of such student creativity.
Consider slightly modifying the activity by pricing the given materials and adjusting the contest objective to which protein works the best and costs the least.
Science Background
The human body is 16% protein. These proteins do many things: they work as enzymes to increase chemical reactions; they store and transport substances; they make up channels in cell membranes to permit specific substances to go in and out of cells; they copy genes to make more proteins; they keep the body well by destroying bacteria; they make up our muscles to enable movement; they transmit messages, and they are what we see when we look at a person’s skin, nails and hair. Proteins use a combination of 20 different amino acids to form different shapes to perform different functions.
In a process called transcription, DNA makes messenger RNA whose job is to leave the nucleus and travel to the ribosome. The ribosome (made up of rRNA) works together with tRNA in a process called translation, to bring specific amino acids that are combined to form the primary protein structure—a straight chain of amino acids.
Once the primary structure is completed, the secondary structure begins as the protein starts to twist into a corkscrew shape or become more like a folded sheet. The tertiary stage follows as the protein begins to fold even more. The last stage or quaternary stage is when several tertiary staged proteins bond to each other.
If mutations exist in the DNA sequence or errors occur in transcription or translation, various consequences result. Sometimes a protein will not be made. Other times an incorrect protein will be made. Both of these situations result in disease.
Engineering Design Process
Engineers follow the steps of the design process to help them solve problems. Each step guides engineers in researching, developing and revising solutions. The steps are typically presented in a circular order, with numerous repetition of steps (iterations) as engineers continually work to improve and evolve design ideas in order to achieve end products that that best meet the original challenge requirements and constraints.
Before the Modeling Structural Proteins Challenge 1
- Assemble materials, weigh the books and write the book weight somewhere that students can see it, such as on the classroom board.
- Make copies of the Proteins Have Different Functions in Our Bodies Reference Sheet, Protein Quiz, and Protein Shapes Affect Their Functions: Structural Worksheet, one each per student.
- Familiarize yourself with the NIH Chapter 1: Proteins are the Body's Worker Molecules at https://www.nigms.nih.gov/education/Booklets/The-Structures-of-Life/Documents/Booklet-The-Structures-of-Life.pdf since you will use some of its content as a resource and visual aid for protein structure. The following four sections are the most helpful for this activity: Proteins are Made from Small Building Blocks, Proteins in All Shapes and Sizes, Small Errors in Proteins Can Cause Disease, and Provocative Proteins.
- Decide if you want (or have time) to have students (or small groups) each research in advance 1) the diseases that results from protein mutations and 2) protein types and their functions. Have students share what they learn with the rest of the class as prompted in the Introduction/Motivation section.
- Make available enough floor space so teams have room to create and test their protein models. They need to build the models on the floor so they have enough room above them to accommodate a tall and heavy stack of books/weights for strength testing, with the first book positioned at 1.5-2 inches above the ground (see Figure 2).
Day 1 with the Students: Protein Shapes Affect Their Functions—Structural
- Present the Introduction/Motivation section to the class. This includes going over protein types using the reference sheet and/or website (nine basic types), talking about what happens if protein mutations occur, administering the pre-quiz, and setting up the (hypothetical) engineering challenge scenario. The engineering design challenge is to create proteins to replace the defective proteins in the child’s body, one for strength, one for capturing oxygen molecules and one for capturing bacteria.
- Explain to the class that the basic idea behind constructing proteins is that first the amino acids are put together in a particular order and then the protein bends and folds into particular shapes. Using the NIH Chapter 1 website at https://www.nigms.nih.gov/education/Booklets/The-Structures-of-Life/Documents/Booklet-The-Structures-of-Life.pdf, discuss the following three sections with the class: Proteins are Made from Small Building Blocks, Proteins in All Shapes and Sizes, and Small Errors in Proteins Can Cause Disease.
- Hand out the structural worksheet and divide the class into student pairs. Direct students to answer the Part A questions, either working as a class or individually, using information provided at the Learn Genetics website (see URL on the worksheet).
- Continue with worksheet Part B; either read the hypothetical scenario and engineering challenge together or independently. Step 1 brainstorming can be done individually, in groups or as a class. Encourage students to share ideas. A helpful prompt is to suggest that they think of shapes and building structures that are strong (such as cylinders, columns and triangles).
- For worksheet step 2, have each student refer to the materials list and come up with a protein design. Once all or most students have some type of labeled drawing or diagram, have students explain their ideas with a partner.
- For worksheet step 3, addressing the design requirement that the protein model must hold a book 1.5-2 inches above the ground, have students discuss their model protein structure ideas with their partners and come up with a design they want to build and test.
- Remind students to clearly label their drawings so that another person would be able to build from them.
- If possible, have students draw three-dimensionally or from different angles. Refer students to the Engineering Design Drawing Rubric for expectations.
- Remind students that their final designs will be different than their initial designs—that’s how the engineering design process works. With open-ended challenges, many possible good solutions could be created the meet the project requirements. The approach is to learn how to design, test and redesign to improve the initial design, to develop it into a great solution.
- Once students have finished initial design drawings, move to step 4. Critique the drawings for detail, using the rubric if desired. Have students write down how much weight they think their protein models might hold; then initial the worksheet. See Figure 1 for an example student-designed structural protein model.
- For step 5, students build their models—after a reminder (on the worksheet) to do their testing on the floor. As they build. suggest they use a few pre-weighed books to make initial assessments of the stability/strength of the model proteins. As you watch the students doing practice testing, notice whether they stack the books uniformly and carefully or not, in order to inform your decision to let students stack their own books during the “official” testing or do it yourself.
- During step 6, the official testing stage, have each group stack books on top of its model protein (see Figure 2). Realize that the physical stacking of the books/weights can be another variable in the protein’s efficiency in meeting the design objective. If students were sloppy stackers during the practice testing, have the instructor do the stacking. Post each group’s test results. Have students record all group data on the worksheet and answer the step 6 worksheet question to evaluate their protein models for their design strengths and weaknesses.
- Next, students move to the redesign stage—deciding how to improve the protein model. They draw new drawings and diagrams, make revised models, and retest, as guided by worksheet steps 7 and 8.
- Direct students to examine and graph the data for all teams. Which model was most successful?
- Next, have students share and compare what worked well, what they changed and what they learned.
- Then have them answer the questions to complete worksheet steps 9 and 10. The concluding worksheet question asks: When DNA has errors in it (mutations that cause disease), it produces faulty proteins or no proteins. If your structure was really a collagen protein that strengthens bones, would it be able to cure the child with brittle bones? Would it have any limitations to the size to which the child could grow? Or did you simply make another mutated structural protein? Use your knowledge of how proteins are constructed to explain.
Before the Modeling Transport Proteins Challenge 2
- Gather materials and make copies of the Protein Shapes Affect Their Functions: Transport Worksheet, one per student
- Set up a three-stage testing area composed of an “oxygen” dispenser, an oxygen-dispensing station (lungs) and an oxygen-dumping station (cells). Oxygen is represented by mini marshmallows.
- Create an “oxygen dispenser,” such as the examples shown in Figure 3. One method is to cut holes in one side of a rectangular gift box so as to permit just a few mini marshmallows to be shaken out at a time. Another method is to tape paper strips across the short dimension of the top opening of an empty rectangular-shaped cardboard tissue box.
- Create an “oxygen-dispensing station” composed of a big box to catch stray marshmallows that do not make it into the student-designed protein models. Into this big box, place a smaller box (or a piece of wood or a book) to serve as an elevated platform to place the model for testing (see Figure 4). Write “LUNGS” on a piece of paper and tape it to the side of the big box.
- On yet another box, the oxygen dumping box, tape a piece of paper on which you have written “CELLS.” After the instructor has counted the oxygen (number of mini marshmallows) they captured, students dump into this last box all the marshmallows from their protein models.
- (optional) Consider increasing the competition by setting up an obstacle course between the oxygen-dispensing stage and the oxygen dumping box. To do this, after each model is tested and its captured oxygen counted, time the team as it transports (through the obstacle course) and dumps (into the “cells” box) its oxygen to see which team is the quickest. For example, require students to run, jump/hop and go under and around an obstacle course of desks and trash cans. This also has design implications if it is explained from the beginning of the challenge as a design consideration. If the transport stage is anticipated to cause the model protein to be jiggled around (as it is carried through an obstacle course), the best designs will have designed the protein model to keep the marshmallows from bouncing out of their compartments too easily. If you include an obstacle course, do the oxygen counting at the end of the course (in the cells box). Make the obstacle course as easy, challenging or competitive as you want.
Day 2 with the Students: Protein Shapes Affect Their Functions—Transport
- Refresh students on the hypothetical engineering design scenario: As engineers, your task is to help a child who has three different diseases. Challenge 1 tested your ability to “cure” the brittle bones of the child. In that challenge (yesterday), some teams “cured” the child by designing very strong proteins while some of the structural protein models ended up being “mutated.” Remember: Your model protein’s function was determined by its shape, which you made when you put together your amino acids (materials).
- Hand out the transport worksheet and organize the class into student pairs.
- To start Part A, direct students to return to the Learn Genetics website (see URL on the worksheet) and answer worksheet Part A questions 1 and 2, either as a class or individually.
- When completed, direct them to the second website (see URL on the worksheet), the online Boundless Biology textbook, to answer worksheet questions 3-6.
- Discuss student answers to question 6 to make sure they understand that even though the three proteins (shown in the website graphic) carry oxygen, they need not be the same shape.
- Moving on to Part B, explain that today’s challenge involves designing an oxygen-carrying molecule called hemoglobin, as described on the worksheet.
- Guided by the step 1 worksheet questions, students brainstorm about the many different ways that “things” are transported, helping them generate ideas that could be applied to the protein design challenge.
- Moving on to step 2, go over the design requirements and constraints. Make sure students understand that they are making many hemoglobin “compartments” that can each hold four oxygen (marshmallows). Their hemoglobin models must catch, hold, transport (be carried) and release oxygen. If you have incorporated an (optional) obstacle course, mention it to students at this time so they can consider its possible design implications.
- Have students draw and label their designs. Remind them to estimate how many oxygen they expect to be able to transport (step 3). Then check their drawings before permitting them to begin building. As they build (step 4), make sure teams use (not eat!) four mini marshmallows to practice testing their hemoglobin models for size and ease of loading/unloading. See Figure 5 for an example student-designed transport protein model.
- Once the hemoglobin protein models are ready to test (step 5), have each team place its model on the oxygen-dispensing platform. Then the instructor uses the oxygen dispenser to shake out the mini marshmallows as uniformly as possible (see Figure 4), with the uncaught oxygen falling into the big LUNGS box. Note: If students shake out their own marshmallows, they usually cannot help but try to fill each hemoglobin and thereby distort the test results.
- After dispensing the oxygen, inspect the protein model for extra oxygen that might have been captured (only four per hemoglobin compartment are permitted)—and remove them.
- Next, students carry the protein model to the CELLS box and dump out the marshmallows. (optional) If an obstacle course has been arranged, time the team as it navigates the obstacle course with its loaded protein model to see which group gets its safely transported oxygen (marshmallows) dumped the quickest.
-
At the CELLS box, students count and record the number of permitted captured marshmallows they dumped into the box. Remind students to record their results on the worksheet along with their reflections on the model’s effectiveness.
-
Then empty the cells box of marshmallows and resupply the dispenser box before the next team’s model testing.
-
Post each group’s test results. Remind students to record the data for all teams’ results on their worksheets.
-
Students continue with steps 6-7—redesigning, building, and testing again, including recording new data and evaluating their revised attempts.
-
Direct students to examine and graph the data for all teams. Which model was most successful?
-
Have students share and compare their protein model designs and results across all teams, then concluding challenge 2 by answering the steps 8 and 9 worksheet questions.
Before the Modeling Defense Proteins Challenge 3
- Gather materials and make copies of the Protein Shapes Affect Their Functions: Defense Worksheet, one per student.
- Cross-shred a supply of paper from the recycling bin. Section off a ~2 x 4-foot area on the floor to use for testing. Spread out the shredded paper in this area. See Figure 6.
Day 3 with the Students: Protein Shapes Affect Their Functions—Defense
- Remind students of the previous two challenges and how some protein models ended up being “mutated” while others were fully functioning due to successfully designed protein shapes.
- Hand out the defense worksheet and sort the class into student pairs.
- To conduct their pre-challenge research, direct students to go to the Learn Genetics website and answer the worksheet Part A questions, either working as a class or individually.
- Moving on to Part B, explain the challenge, as described on the worksheet. Guided by the step 1 worksheet questions, students brainstorm about generally how “things” are captured or attacked. If students suggest attacking with methods like fire, heat and acid, steer them in another direction by asking how the protein might be designed to capture bacteria before it melts or dissolves the bacteria.
- Once students have brainstormed, considered the requirements and constraints, drawn designs, and had them teacher-approved, direct them to build the models (steps 2-4).
- To test the efficiency of the protein models (step 5), have each group use its model to “capture” as much bacteria (shredded paper) as possible from a layer of shredded paper on the floor in a 2 x 4-foot area. Either set a time limit or let students get as much paper on their protein as possible.
- To measure success, collect data for each model by massing the shredded paper each model picks up, which may be done a few different ways.
- Weigh the model before and after testing. The difference is the mass of paper picked up.
- Weigh the shredded paper before placing it on the floor. Weight the amount left on the floor after the model has done its work to capture the paper. The difference is the mass of paper picked up.
- Since paper pieces tend to fall off the model as it is picked up off the floor, decide whether or not to count those falling pieces towards the total mass. If you want that paper to count, have teams immediately place their models in a bag or on a tray before carrying it to the scale.
- Remind students to record the data for all teams’ results on their worksheets, and answer the step 5 reflection questions.
- Students continue with steps 6-7—redesigning, building, and testing again, including recording their new data and evaluations of the revised attempts. If they used a lot of tape in their designs, they may need another roll, or to completely rebuild their models from scratch.
- Direct students to examine and graph the data for all teams. Which model was most successful?
- Then lead a class discussion so teams can share and compare what worked well for their defense model designs, what they changed and what they learned.
- Then have students answer the steps 8 and 9 questions to complete the worksheet.
Vocabulary/Definitions
amino acid: An organic (carbon-based) macromolecule compound that contains carbon and nitrogen, which are the building blocks of protein, and are made by living plant or animal cells or are obtained from the diet.
defense protein: A protein that is assembled and folded in such a way that enables it to defend the body against invaders such as bacteria.
macromolecule: A very large molecule, such as protein. Commonly created by the polymerization of smaller subunits (monomers).
protein: A large biomolecule or macromolecule consisting of one or more long strings of amino acids folded in particular shapes to perform a wide variety of specific functions in the body of organisms. Proteins differ from one another mainly in the sequence of amino acids, which results in proteins folding into specific 3D structures that determine their functionalities.
ribosome: A cell organelle with the function to link together amino acids to make proteins.
structural protein: A protein that is assembled and folded in such a way to provide structure and support in the body, such as the collagen in bones.
transport protein: A protein that is assembled and folded in such a way to carry and move molecules within the body, such as how hemoglobin in the blood carries oxygen.
Assessment
Pre-Activity Assessment
Protein Pre-Quiz: After presenting the Introduction/Motivation section content, administer the seven-question short-answer Protein Pre-Quiz to verify students’ understanding of proteins.
Brainstorming: Each challenge asks students think about the function of that particular protein type, the amino acids (building materials) they will use and how to shape them accordingly. Either have students brainstorm in small groups or as a class.
Activity Embedded Assessment
Worksheets: For each challenge, students follow along and answer questions related to brainstorming, designing, planning, building, testing, evaluating and redesigning/retesting their three different protein types. In each case, a worksheet guides them through the steps of the process. Review their worksheet answers, data, graphs and explanations to gauge their engagement and depth of comprehension.
Post-Activity Assessment
Final Presentations and Reflections: After testing their protein models, students share, compare and discuss what they observed as their design strengths and weaknesses. They highlight the properties of their models that enable them to do a better job (or not as good a job) as other groups’ protein models. When DNA has errors in it (mutations that cause disease), it produces faulty proteins or no proteins. Have students explain and describe how amino acids come together to make proteins and how the shape of the protein is related to its function. For each challenge, expect students to be able to evaluate and come to a conclusion about whether or not their placement of particular “amino acids” and their subsequent shaping resulted in effective proteins that met the requirements for each particular protein type.
Investigating Questions
Suggested questions to ask students while they are designing and building their protein models.
General: Are you building your protein to the specifications you drew? If you’ve made adjustments to your prototype, have you made changes on your drawing? Are you using your materials wisely?
For the strength challenge: Are you taking into consideration that many books will be stacked on this model during testing? Will books easily balance on your structure? Have you measured to be sure the books will be 1/5–2 inches above the floor? Have you placed a book on your model to test it to make sure it will hold at least one book?
For the transport challenge: Since only four oxygen will be counted for each hemoglobin compartment, have you maximized the number of compartments your protein model contains? Will the oxygen be able to get into and out of each compartment easily? How will you keep the oxygen from bouncing out when you transport it from the lungs to the cells?
For the defense challenge: Will your entire protein model be moving or are only parts of it moving? If so, which parts? What will you use as a handle? Are you making sure all antibody parts are connected?
Safety Issues
For the structural proteins challenge, be sure students test their proteins on the floor! Since it is possible that these protein models could hold 150 lbs or more, you want to avoid having the test load crash down on students or other objects (and possibly damage the textbooks).
For the transport proteins challenge, be aware that some students may have allergies to mini marshmallows. Use your judgement to substitute with alternate items such as cotton balls or bingo chips.
Troubleshooting Tips
Some students have ideas right away and some don’t. Remind them that this is a process and until they first test their protein models, they won’t know how well they work. So just get started and improve from there. The idea is to create and test as a way to improve their original protein model ideas. Encourage students to work through each step of the engineering design process as they create their models.
Activity Scaling
- To simplify for lower grades, just focus on one protein type, such as the structural protein.
- For higher grades, have students consider cost analysis between the cost of materials used and model success based on testing results across the teams.
Additional Multimedia Support
Basic Genetics. Learn.Genetics, Genetic Science Learning Center, University of Utah. https://learn.genetics.utah.edu/content/basics/
Chapter 1: Proteins are the Body’s Worker Molecules. 2011. The Structures of Life, Science Education, National Institute of General Medical Sciences, National Institutes of Health, U.S. Department of Health and Human Services, Bethesda, MD. https://www.nigms.nih.gov/education/Booklets/The-Structures-of-Life/Documents/Booklet-The-Structures-of-Life.pdf
Components of the Blood > The Circulatory System. In Boundless Biology (online textbook). Lumen Learning Courseware, LumenCandela. https://courses.lumenlearning.com/boundless-biology/chapter/components-of-the-blood/
Types of Proteins. Learn.Genetics, Genetic Science Learning Center, University of Utah. https://learn.genetics.utah.edu/content/basics/proteintypes/
Subscribe
Get the inside scoop on all things TeachEngineering such as new site features, curriculum updates, video releases, and more by signing up for our newsletter!More Curriculum Like This

Students learn about mutations to both DNA and chromosomes, and uncontrolled changes to the genetic code. They are introduced to small-scale mutations (substitutions, deletions and insertions) and large-scale mutations (deletion duplications, inversions, insertions, translocations and nondisjunction...

As a class, students work through an example showing how DNA provides the "recipe" for making human body proteins. They see how the pattern of nucleotide bases (adenine, thymine, guanine, cytosine) forms the double helix ladder shape of DNA, and serves as the code for the steps required to make gene...

Students are introduced to the latest imaging methods used to visualize molecular structures and the method of electrophoresis that is used to identify and compare genetic code (DNA).
Copyright
© 2018 by Regents of the University of Colorado; original © 2017 North Dakota State UniversityContributors
Lauren Sako; Beth PodollSupporting Program
RET Program, College of Engineering, North Dakota State University FargoAcknowledgements
This curriculum was developed in the College of Engineering’s Research Experience for Teachers: Engineering in Precision Agriculture for Rural STEM Educators program supported by the National Science Foundation under grant no. EEC 1542370. Any opinions, findings, and conclusions or recommendations expressed in this material are those of the author(s) and do not necessarily reflect the views of the National Science Foundation.
Special thanks to Alan Kallmeyer and Bradley Bowen.
Last modified: August 16, 2023
User Comments & Tips