Quick Look
Grade Level: 11 (9-12)
Time Required: 1 hours 30 minutes
(can be split into different sessions)
Expendable Cost/Group: US $0.00 This activity also uses one or two non-expendable (reusable) air quality monitors, which can be rented; see the Materials List and Other sections for details.
Group Size: 28
Activity Dependency:
Subject Areas: Earth and Space, Measurement, Science and Technology
NGSS Performance Expectations:
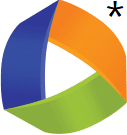
HS-ESS3-1 |
Summary
As a class, students use a low-cost air quality monitor (a rentable “Pod”) to measure the emissions from different vehicles. By applying the knowledge about combustion chemistry that they gain during the pre-activity reading (or lecture presentation, alternatively), students predict how the emissions from various vehicles will differ in terms of pollutants (CO2, VOCs and NO2), and explain why. Combustion is a common burning reaction that occurs often in the world around us and that we look at when studying air quality because the reaction emits many different common pollutants. After data collection, students examine the time series plots as a class—a chance to interpret the results and compare them to their predictions. Short online videos and a current event article help to highlight the real-world necessity of understanding and improving vehicle emissions. Numerous student handouts are provided. The activity content may be presented independently of its unit and without using an air quality monitor by analyzing provided sample data.Engineering Connection
This activity walks students through a test and analyze experience that mirrors the experiences of engineers who design cars and engineers who regulate their emissions. Industrial, mechanical and electrical engineers work together to design the most efficient and well-functioning cars. It is important to continually advance automotive technology in order to improve efficiency and safety, and decrease environmental impact. Emissions are regulated to ensure that car companies continue to improve automotive technology.
The activity begins with background information on combustion chemistry, obtained either by a reading or slide presentation, including extending these concepts to vehicles, covering how vehicle operation/design and control technologies impact combustion, and thus emissions. This shows students an example of engineers applying scientific concepts to automobile design. During data collection, students carry out a simplified version of what regulatory agencies do—measuring vehicle emissions and interpreting the data. Students are also tasked to critique the design of the data collection procedures and discuss ways to ensure consistent data collection processes from vehicle to vehicle.
Learning Objectives
After this activity, students should be able to:
- List the products of complete and incomplete combustion and explain what physical and chemical characteristics lead to the different outcomes.
- Provide examples of how changes made by engineers over time to vehicle designs and control technologies have reduced the emission of harmful pollutants.
- Interpret emissions data from a plot, using concepts from combustion chemistry and vehicle characteristics to support the explanation.
- Use the Pods (air quality monitors) to collect data on their own.
Educational Standards
Each TeachEngineering lesson or activity is correlated to one or more K-12 science,
technology, engineering or math (STEM) educational standards.
All 100,000+ K-12 STEM standards covered in TeachEngineering are collected, maintained and packaged by the Achievement Standards Network (ASN),
a project of D2L (www.achievementstandards.org).
In the ASN, standards are hierarchically structured: first by source; e.g., by state; within source by type; e.g., science or mathematics;
within type by subtype, then by grade, etc.
Each TeachEngineering lesson or activity is correlated to one or more K-12 science, technology, engineering or math (STEM) educational standards.
All 100,000+ K-12 STEM standards covered in TeachEngineering are collected, maintained and packaged by the Achievement Standards Network (ASN), a project of D2L (www.achievementstandards.org).
In the ASN, standards are hierarchically structured: first by source; e.g., by state; within source by type; e.g., science or mathematics; within type by subtype, then by grade, etc.
NGSS: Next Generation Science Standards - Science
NGSS Performance Expectation | ||
---|---|---|
HS-ESS3-1. Construct an explanation based on evidence for how the availability of natural resources, occurrence of natural hazards, and changes in climate have influenced human activity. (Grades 9 - 12) Do you agree with this alignment? |
||
Click to view other curriculum aligned to this Performance Expectation | ||
This activity focuses on the following Three Dimensional Learning aspects of NGSS: | ||
Science & Engineering Practices | Disciplinary Core Ideas | Crosscutting Concepts |
Construct an explanation based on valid and reliable evidence obtained from a variety of sources (including students' own investigations, models, theories, simulations, peer review) and the assumption that theories and laws that describe the natural world operate today as they did in the past and will continue to do so in the future. Alignment agreement: | Resource availability has guided the development of human society. Alignment agreement: Natural hazards and other geologic events have shaped the course of human history; [they] have significantly altered the sizes of human populations and have driven human migrations.Alignment agreement: | Empirical evidence is required to differentiate between cause and correlation and make claims about specific causes and effects. Alignment agreement: Modern civilization depends on major technological systems.Alignment agreement: |
Common Core State Standards - Math
-
Model with mathematics.
(Grades
K -
12)
More Details
Do you agree with this alignment?
-
Reason abstractly and quantitatively.
(Grades
K -
12)
More Details
Do you agree with this alignment?
-
Summarize, represent, and interpret data on two categorical and quantitative variables
(Grades
9 -
12)
More Details
Do you agree with this alignment?
-
Represent data on two quantitative variables on a scatter plot, and describe how the variables are related.
(Grades
9 -
12)
More Details
Do you agree with this alignment?
International Technology and Engineering Educators Association - Technology
-
Students will develop an understanding of the effects of technology on the environment.
(Grades
K -
12)
More Details
Do you agree with this alignment?
-
Critue whether existing and proposed technologies use resources sustainably.
(Grades
9 -
12)
More Details
Do you agree with this alignment?
-
Assess a technology that minimizes resource use and resulting waste to achieve a goal.
(Grades
9 -
12)
More Details
Do you agree with this alignment?
State Standards
Colorado - Math
-
Summarize, represent, and interpret data on two categorical and quantitative variables.
(Grades
9 -
12)
More Details
Do you agree with this alignment?
-
Represent data on two quantitative variables on a scatter plot, and describe how the variables are related.
(Grades
9 -
12)
More Details
Do you agree with this alignment?
Colorado - Science
-
Matter can change form through chemical or nuclear reactions abiding by the laws of conservation of mass and energy
(Grades
9 -
12)
More Details
Do you agree with this alignment?
-
Predict reactants and products for different types of chemical and nuclear reactions
(Grades
9 -
12)
More Details
Do you agree with this alignment?
-
The interaction of Earth's surface with water, air, gravity, and biological activity causes physical and chemical changes
(Grades
9 -
12)
More Details
Do you agree with this alignment?
-
Analyze and interpret data, maps, and models concerning the direct and indirect evidence produced by physical and chemical changes that water, air, gravity, and biological activity create
(Grades
9 -
12)
More Details
Do you agree with this alignment?
Materials List
Before the activity, each student needs:
- pen or pencil
- Pre-Activity Reading (alternative: watch the Pre-Activity Presentation presented by the teacher)
- Pre-Activity Problem Set
- Vocabulary List
Each group needs:
- pens or pencils
- Activity Data Sheet, one per student
- Results and Reflection Questions, one per student
- (optional) Current Event Article or Engineering Identities Worksheet, one per student
- Connecting to the Big Picture Handout, one per student
To share with the entire class:
- 1-2 air quality monitors, such as a rented AQ-IQ Kit (containing 2 Pod low-cost air quality monitors) from the University of Colorado Boulder, or other low-cost monitors with multiple gas-phase sensors; see the Other section for details on obtaining the Pod (or alternate) monitors
- box or plastic container on which to position the monitor near the tailpipes (see Figure 3)
- watch, to measure test times
- ruler, to measure tailpipe-to-monitor distances
- 2-4 vehicles, from which to test exhaust emissions; it is best if the vehicles differ in engine size, fuel type, age and/or condition
- a computer/projector system, for the teacher to show one or two online videos, show the (optional) Pre-Activity Presentation, and plot the data for the class discussion
Worksheets and Attachments
Visit [www.teachengineering.org/activities/view/cub_airquality_lesson01_activity2] to print or download.Pre-Req Knowledge
Basic chemistry knowledge; it is helpful if students have seen simple reactions and understand the notation used in chemical formulas.
The air quality monitors were introduced in the first lesson and activity in this unit, but you may want to practice using the monitors once more before this activity. Information on obtaining monitors is available in the Other section.
Introduction/Motivation
When it comes to controlling pollution from vehicles and regulating the fossil fuel industry (coal, oil, natural gas), we try to strike a balance between limiting pollutants and cost. The more that we use vehicles that are dependent on fossil fuels, the more pollutants that we disperse into our atmosphere, damaging our environment and ourselves. Switching from fossil fuels to other sustainable sources of energy requires money to develop the technology and implement the infrastructure necessary to transfer to a different system. As a society, we aim to balance the amount of pollutants that we disperse into our atmosphere with the cost of developing and implementing new technology.
Let’s consider the costs and benefits. What are some of the costs to humans of using vehicles that exhaust pollutants into the air? (Have students list their thoughts on the classroom board or verbally share their ideas. Cost examples: Pollutants cause poor air quality, which negatively impacts public health, contributes to climate change, degrades the environment, alters ecosystems for plants and animals, and depletes the supply of non-renewable fuels.) And what are some of the benefits? (Benefit examples: Vehicles provide transportation for people, food and goods with relative ease, and a robust industry that contributes to the economy via manufacturing, energy extraction, and other directly related jobs. These are just a few examples; students may think of more.)
So, as you can see, the use of vehicles affects us at many different levels and in many different positive and negative ways. This is often the situation with technologies that our society relies on and it is up to not only engineers, but also scientists, regulators and politicians to work towards maximizing the benefits of a technology while minimizing the negative consequences. We have seen this in the automobile industry during the past 40 years with many changes being implemented such as better control technologies, like catalytic converters (which are emissions control devices that convert CO to CO2), the removal of lead from gasoline (lead is a neurotoxin that used to be a gasoline ingredient), and increased fuel efficiency.
However, we still have much improvement to make since the use of fossil fuel vehicles continues to increase globally (in this case, we are referring to gasoline-powered cars), particularly in developing countries without the regulations in place that countries like the U.S. have established. To protect public and environmental health, the U.S. has regulations on CO, particulate matter and NOx emissions from vehicles. Without those vehicle emissions regulations, we would have many more problems with smog, air quality and other air pollutants in cities across the country.
Let’s take a look at some real-world examples of how science, technology and society intersect around the transportation industry. (Show students the suggested video listed below. As an alternative, have students read the Current Event Article and answer/discuss its questions.)
- Petrol and diesel car sales ban brought forward to 2035, BBC News.
An explanation of the proposed ban in the UK on selling new petrol, diesel or hybrid cars to curb the negative effects of climate change. See: https://www.bbc.com/news/science-environment-51366123
- Volkswagen’s Diesel Scandal, Explained (2:30 minutes), The Verge, YouTube.
An explanation of the “defeat device” in the programming of many Volkswagen-made diesel cars that makes it look like the cars meet emissions standards when they don’t. The company is in trouble for illegally getting around the emissions restrictions, which put as much as 40 times more pollutants in the air than permitted. Unburned fuel and nitrogen oxide pollutants are mentioned. See: https://www.youtube.com/watch?v=CQ4irwe3ZDk
Today, you will learn about the scientific principles that determine what comes out of a tailpipe, and what factors can change or influence this. You will also practice making your own emissions measurements and interpreting the resulting data. These factors are a big consideration for car industry engineers. Mechanical, chemical, electrical and many other kinds of engineers work together in the automotive industry to improve the efficiency and performance of cars.
Procedure
Background Information (also provided in Pre-Activity Presentation and Reading)
According to the Inventory of U.S. Greenhouse Gas Emissions and Sinks, in 2018, transportation accounted for 28% of greenhouse gas emissions [1]. Since transportation is such a major contributor to poor air quality and climate change, it is important that we monitor and study emissions from all forms of transportation.
Measurements of emissions from transportation are made in a variety of ways: individual cars undergo testing, monitors are placed on roadways (or interstate on-ramps), and data analysis from stationary monitors can sometimes provide an idea of how much of each pollutant comes from vehicles vs. industry (which is called source apportionment).
Combustion Chemistry
Combustion is a chemical reaction between oxygen and a hydrocarbon that produces water, heat and carbon dioxide. Combustion in vehicles involves burning a carbon-based fuel in the presence of air (oxygen and nitrogen), which results in the production of energy and combustion by-products. Fuel is represented by the formula CxHy, which represents x number of carbons and y number of hydrogens. C8H18 is average gasoline and C12H23 is the average formula for diesel. A different composition of fuel (different numbers of C and H) results in different physical properties. For example, diesel fuel has a higher energy content than gasoline. For comparison, these energy contents are listed below. Note that BTU is the acronym for British thermal unit, which is a measure of work equivalent to the amount of heat it takes to raise the temperature of 1 pound of water 1 °F.
- Gasoline energy content = 122,364 BTU/gal
- Diesel energy content = 138,490 BTU/gal
Reaction 1: Complete Combustion CxHy + O2 → CO2 + H2O
Complete combustion occurs when enough oxygen is present to fully combust each carbon in the fuel, or enough oxygen to provide two oxygen molecules for each carbon molecule. The by-products of complete combustion are water vapor and carbon dioxide. Since carbon dioxide is not a concern for human health, this type of combustion is (generally) the cleanest. Although CO2 is not bad for human health (we breathe it out with every breath!), it is a greenhouse gas and increased concentrations are bad for the environment.
Reaction 2: Incomplete Combustion CxHy + O2 → CO + VOCs + CO2 + H2O
Combustion is incomplete when not enough oxygen is present to completely combust the fuel supply. As a result of the lack of oxygen, by-products like CO and VOCs are created. Carbon monoxide is harmful to human health and VOCs can be harmful depending on the compound. Carbon dioxide (CO2), along with CO, VOCs, and H2O, are by-products of incomplete combustion.
Reaction 3: Thermal NOx Formation N2 + O2 → NO + NO2
This reaction does not involve a direct interaction with the fuel. Thermal NOx formation is the result of high-temperature combustion. At high temperatures, the oxygen (O2) and nitrogen (N2) in the air are split and bond to one another, creating NOx (including both NO and NO2). NOx is a concern for human health.
Internal Combustion Engines and Air-Fuel Ratios
The air-fuel ratio is the relative amounts of air and fuel available to the engine for combustion, and can affect how internal combustion engines perform in vehicles. The air-fuel ratio impacts how much energy is produced and what combustion by-products are released. For comparison purposes, Table 1 lists the advantages and disadvantages of the fuel-rich and fuel-lean combustion scenarios. Basically the process is as follows:
- Fuel rich means there is more fuel and less air, which results in more power, but also more incomplete combustion by-products.
- Fuel lean means there is less fuel and more air, which results in a more complete combustion, but less power.
The Figure 1 plot shows how pollutants can vary with different air-fuel ratios. Observations when looking at the Figure 1 emission curves:
- CO is the highest on the fuel-rich side.
- NOx is the highest when we have the “best economy” or when we are using the fuel most efficiently. This means we also have the most effective and complete combustion, thus the highest temperatures.
- VOCs are high both on the fuel-rich side (when not enough O2 is present to combust the fuel) AND when we are very fuel-lean (in this case, fuel is being heated, thus increasing volatilization, but the fuel is not combusting).
- If we were to add CO2, we would also have the most CO2 when we have the best fuel economy.
Control Technologies
The air-fuel ratio is also determined by effective operation of control technologies. One well-known control technology is the catalytic converter (see Figure 2). This device, attached to cars, uses oxidation and reduction reactions to convert harmful pollutants (CO, VOCs, NOx) to gases that are not dangerous to human health (CO2 and water vapor). Catalytic converters first became widely used in the 1970s to meet stricter emissions regulations set by the U.S. EPA. Other strategies that have improved air quality during the past 40 years include removing the lead from gasoline and adding additional control technologies to diesel vehicles such as filters to capture the high particulate matter in the exhaust.
Notes on the Air Quality Monitors (Pods)
The following information is especially helpful if you have not used the Pods before and a refresher if you have. See the Other section for information about how to rent an AQ-IQ Kit that contains two Pods (air quality monitors).
- The monitors use electronic sensors. That means that you turn it on, the sensors run continuously, and the circuit board records data periodically to the device’s SD card.
- The recorded data is in the form of voltages, so you can analyze the raw signal for trends (which we do in this activity) or convert the data into concentrations. The latter can be done using different types of calibration; information on calibration is available on the project website and in the user manual. Converting data using global equations is done automatically using the data tools developed for the Pods.
- The electronic sensors need time to “warm-up,” or stabilize and reach an equilibrium of sorts, which takes about 30 minutes and is achieved by simply plugging in the Pod, turning it on and letting it sit.
- “Baseline measurements” are necessary to differentiate the data from each pollution source (or from each vehicle, in this case). We use the term “baseline” because it describes conditions without the added source. Many of the pollutants we are measuring are already present in the air, but at lower concentrations. Thus, the data we collect (according to the activity’s Procedure instructions) will report the baseline conditions (what the is air like without the source we are examining), then the impact of adding a source, and then the baseline again so we can see what happens when we remove the first source before adding a second source (or testing a second vehicle).
- Again, the AQ-IQ Kit includes everything needed to use the monitors, including power cords, batteries and a user manual.
- If any problems arise with the collected data or plotting, OR if this content is being presented as a standalone activity without the availability of an air quality monitor, use the Sample Data file as an alternative plotting from which to complete the activity (see further elaboration in in the Procedure section.)
Before the Activity
- Gather materials and make copies of the Pre-Activity Problem Set, Pre-Activity Reading, Vocabulary List, Activity Data Sheet, Results and Reflection Questions, (optional) Current Event Article or Engineering Identities Worksheet and Connecting to the Big Picture Handout.
- The day before conducting the activity, assign students the Pre-Activity Reading and Pre-Activity Problem Set as background preparation homework the night before. Alternatively, have them complete the problem set during or after the 18-slide Pre-Activity Presentation that you present as a class lecture at the beginning of the activity. Note that the latter approach will increase the time requirement to conduct the activity. Both the reading and the presentation (as well as the Teacher Background section) contain the same information about air quality data collection and monitoring emissions, including an explanation of the three main reactions that take place during combustion, the air-fuel ratio, fuel rich vs. fuel lean, and catalytic converters. Note that unique to the slide set is a suggested script and reference information in the slide notes, as well as (the last) four slides with information to help students see the upcoming activity experiment as a conceptual model (a system with inputs and outputs through a process), the overall steps of the experimental procedure, and data sheet questions to consider while tests are going on—slides you may want to show right before and/or during the experiment.
- Have students fill in definitions for each word on the Vocabulary List. Instruct students to keep the list as a resource for this activity as well as the other associated activities.
- Waiting for measurements to complete during the baseline and idling periods can be somewhat tedious. Use the data collection time to have students do all or some of the following to keep them focused and/or generate good class discussions.
- Review and discuss the problem set that students completed the night before
- Read and discuss the Current Event Article, which describes how officials put limitations on the number of automobiles permitted on the road in Paris during very smoggy days
- Complete the Engineering Identities Worksheet, which is intended to open up students’ minds to the broad possibilities of where studying engineering might take them; examining their answers provides you with useful information about their interests.
- Determine which testing vehicles you will use and plan ahead. Make arrangements for them to be parked near each other in a nearby parking lot. It is important that the cars have been off for similar amounts of time. Also, secure any permissions needed to conduct the experiment in a parking lot with students.
- Make sure the air quality monitor battery(ies) are charged (if outlet power is not available near the cars). Make sure the monitor(s) has had 30 minutes to warm up, such as in the classroom during the lecture.
With the Students: Testing and Data Collection
- As a class, review the problem set that students completed as homework, answering any questions.
- Present to the class the Introduction/Motivation content, which includes showing one or two short online videos.
- As a class, review the procedures on the data sheet.
- Discuss variables that may impact students’ data collection and strategies to improve the quality of the data collection process. For example, it is best if the air quality monitor is placed the same distance from each tailpipe; use a ruler to measure this distance so it can be precisely replicated at each vehicle.
- Move all the students and equipment to the parking lot. Tip: Engage students to do as much as possible to make the activity happen, such as carrying equipment, setting up equipment by the tailpipes, and checking to make sure the monitor is working. This is especially important if you are intending to complete all activities in this unit, so that students gain experience and confidence with the equipment.
- As guided by the user manual, set up the Pod near the first vehicle’s tailpipe. An example ideal setup is shown in Figure 3. Then run the tests to gather data, following the data sheet procedures as recapped below. Remember to record the start/stop times.
- Run the air quality monitor for ~5 minutes to collect a baseline, which serves as a measurement of the ambient levels of pollutants.
- Then start vehicle #1 and idle for 3–5 minutes.
- Turn off vehicle #1 and collect 5 more minutes of baseline data, which also gives the sensors time to recover (re-equilibrate).
- Move the equipment setup to vehicle # 2 and repeat both the idling and the post-testing baseline data gathering.
- Either repeat this process multiple times for the same two vehicles or conduct the process for up to four or five vehicles, depending on the time available and class interest.
- During this testing process, have students record data collection information on their data sheets in the Notes and Observations section, and then have students graph their hypotheses on the final page.
- While waiting during the data collection baseline and idling periods, have students read and complete the Current Event Article and/or Engineering Identities Worksheet, and/or lead class discussions about the Pre-Activity Problem Set and/or current event article.
- Conclude with a final baseline measurement and then bring the Pod(s) and students back into the classroom.
With the Students: Data Plotting and Analysis
- Plot the data. (Refer to the Pod’s user manual for instructions and tools available to assist with this process.) If any problems arise with the data or plotting, hand out the Sample Data (which matches Figure 4) as an alternative from which to discuss what data from this activity would look like.
- As a class, one pollutant at a time, examine and discuss the results. Then, consider how the different emission profiles vary from vehicle to vehicle. See Figure 4 as an example (which is reproduced larger on page 2 of the sample data handout). Below are examples of the types of observations to mention and discuss when analyzing the time series plots (using the Figure 4 plots as an example):
- CO2: Notice that each of the four tests shows a clear response from the CO2 sensor, with the magnitude larger for the truck than the car. Also, the flat line during the second test indicates the sensor has maxed out.
- NO2: Similarly, we see some NO2 sensor response during each test, with the most from the truck.
- VOC sensor 1 (lighter hydrocarbons): Notice an interesting spike at the beginning of each test with the truck, which likely indicates the presence of volatiles that accumulated in the engine and were pushed out upon ignition; and then the amount of VOCs is less during the rest of the idling period.
- VOC sensor 2 (heavier larger hydrocarbons): Notice that the difference between the car and the truck is more apparent for this sensor, indicating that the truck is releasing a fair amount more of heavier hydrocarbons.
- Overall: In general, this data indicates that the truck exhaust has more emissions than the car, which makes sense, given that it is a larger vehicle with a more powerful engine. Additionally, the greater amount of the heavy hydrocarbons suggests that the truck is running more fuel-rich and therefore experiencing more incomplete combustion.
- One last note: For this data, the vehicles were parked parallel to each other, however their tailpipes were located in different positions on the vehicles and thus their exhausts were impacted by the wind differently. This may mean that the emissions from the car were more diluted by the wind than those from the truck, but the amount of this possible impact is unknown. This is known as a “confounding factor.”
- Conclude by having students complete the Results and Reflections Questions.
- Then assign the Connecting to the Big Picture Handout as homework.
Vocabulary/Definitions
air-fuel ratio: A ratio of the relative amounts of air and fuel used in the combustion reaction. The ratio affects the reaction’s by-products. For example, not enough air results in a fuel-rich burn, which corresponds to more incomplete combustion. Alternatively, an air-rich burn results in more complete combustion because no shortage of oxygen molecules exists. Also called the air-to-fuel ratio.
British thermal unit : A unit of work. The amount of heat required to raise the temperature of 1 pound of water 1 degree Fahrenheit. It is equivalent to 1,055 joules. Abbreviated as BTU.
carbon dioxide : A colorless and odorless gas. A gas-phase pollutant. Composed of 1 carbon atom and 2 oxygen atoms. Generated by the respiration of animals and the combustion (burning) of fuels that contain carbon. Abbreviated as CO2.
carbon monoxide: A colorless, odorless and tasteless gas. A compound that is a product of incomplete combustion and is dangerous to human health. Composed of 1 carbon atom and 1 oxygen atom. Abbreviated as CO.
catalytic converter: An emissions control device for cars that converts toxic gases and pollutants to less harmful pollutants. The device takes CO and unburned hydrocarbons and produces CO2, and reduces NOx to less harmful molecules.
combustion : A high-temperature chemical reaction with hydrocarbon and oxygen as the reactants and heat, carbon dioxide and water as the products.
complete combustion: Combustion in which all of the available carbon is fully oxidized to CO2, which requires sufficient oxygen and high enough temperatures for efficient burning. Example: A modern vehicle with no visible emissions leaving the tailpipe, because CO2 is an invisible gas.
confounding factor: A variable that correlates with the dependent and independent variable. For example, wind could be a confounding factor when measuring car emissions because it could affect the measurements.
emissions: The release of pollutants produced during a reaction (in the case of air pollutants, into the atmosphere). For example, exhaust gas from motor vehicles.
fossil fuel: A hydrocarbon material used for fuel that was formed from the natural process of decomposing animal and plant matter. Formed deep under the Earth’s surface under high pressure and high temperature over millions of years. Examples: petroleum, coal, natural gas.
gas-phase pollutant: A compound comprised of multiple atoms (such as carbon dioxide with 1 carbon and 2 oxygens) existing in the gaseous physical phase.
hydrocarbon: A compound that contains only carbon and hydrogen atoms. Another term for VOC. Abbreviated as HC.
incomplete combustion: Combustion in which all available carbon is not completely oxidized and products such as CO, VOCs, and unburned fuel are released. Typically, these emissions chemicals are dangerous to human health. Circumstances that can cause incomplete combustion are lower temperatures and insufficient oxygen. Observed by “rolling coal” aka black smoke billowing from tailpipes.
nitrogen dioxide : A gas-phase compound made of 1 nitrogen atom and 2 oxygen atoms. It is formed during high-temperature combustion from the nitrogen that exists in the air. High-temperature combustion also produces nitrogen monoxide (NO). The sum of the amount of NO and NO2 is the amount of NOx present; in other words NOx is a term that includes both NO and NO2.
ozone: A pale blue gas with a distinctively pungent smell. It is a secondary pollutant formed by NOx and VOCs in the presence of sunlight. Dangerous to human health at ground level, but high in the stratosphere it protects humans from harmful UV rays. Mnemonic: “good up high, bad nearby.”
particulate matter: A microscopic solid or liquid compound that may be natural or human-made. Very small particulate matter may be a conglomerate of gas-phase compounds; larger particulate matter can be dust or pollen.
source apportionment: A type of mathematical analysis on a data set that includes many different pollutants, the result of which provides an idea of how much of each pollutant comes from the different local sources. For example, it might tell you that 70% of CO2 comes from traffic, while 30% comes from a local power plant.
volatile organic compound: An organic chemical that has a high vapor pressure at ordinary room temperature, such that it volatizes (enters the gas phase) at room temperature and pressure. An example is formaldehyde (CH2O, 1 carbon, 2 hydrogens, and 1 oxygen atom). Abbreviated as VOC. VOCs are also gas-phase compounds. VOCs also include products of incomplete combustion (when a carbon-fuel is not completely burned, resulting in only CO2).
Assessment
Pre-Activity Assessment
Problem Set: To provide and then verify that students have adequate background information to conduct the activity, assign them the Pre-Activity Reading and Pre-Activity Problem Set as homework the night before the activity. As an alternative, instead of making the problem set a pre-activity homework assignment, begin the activity by going through the 18-slide Pre-Activity Presentation as a class and having students complete the problem set during and/or after the slides.
Activity Embedded Assessment
Data Sheet: During the experiment, have students use the Activity Data Sheet to guide them in understanding the equipment; conducting the testing procedure; collecting data, measurements and observations; and graphing their hypotheses (predictions) about the emissions data. Review their worksheets to gauge their engagement and depth of comprehension.
Post-Activity Assessment
Results and Reflection: At activity end, after reviewing the results, have students complete the Results and Reflections Questions to summarize their findings and reflect on the activity. Review their answers to assess their takeaways from the activity.
Connecting to the Big Picture: As homework, assign students to complete the Connecting to the Big Picture Handout to help them place what they learned about emissions and air quality monitoring (as applies to vehicles) into a larger context (as applies to fossil fuels and energy use and development). Answering the handout questions also helps them think about topics to which they could tie their later projects in the unit. If possible, take time to discuss their answers during the following class periods.
Safety Issues
While the vehicles are idling, minimize the possibility of inhaling the tailpipe emissions by making sure students stand upwind from them and far enough back (at least five feet) so that you cannot smell the emissions.
Warn students not to touch the vehicle tailpipes since they get hot during idling.
Troubleshooting Tips
If it is really windy, place the Pod closer to the tailpipe (0.5-1 ft.).
If it is a clear still day, place the Pod further from the tailpipe (1-2 ft.).
Additional Multimedia Support
Software to aid in plotting and examining the monitor data is explained in the Pod user manual. All downloads and additional assistance/information are available at https://www.colorado.edu/aqiq/. Alternatively, use Microsoft® Excel® to plot and visualize the data.
Subscribe
Get the inside scoop on all things TeachEngineering such as new site features, curriculum updates, video releases, and more by signing up for our newsletter!More Curriculum Like This

Students learn the basics about the structure of the Earth’s atmosphere, the types of pollutants that are present in the atmosphere (primary, secondary, gas-phase compounds, particulate matter), and the importance of air quality research. They are also introduced to some engineering concepts such as...
References
[1] Sources of Greenhouse Gas Emissions. Climate Change, U.S. Environmental Protection Agency. Accessed August 14, 2020. https://www.epa.gov/ghgemissions/sources-greenhouse-gas-emissions
Other Related Information
AQ-IQ Air Quality Monitor Information
This curriculum was designed to support high school students’ use of a low-cost air quality monitor developed by the Hannigan Lab at the University of Colorado Boulder called a “Pod.” Pods can be rented and shipped from the university; see below for details. Alternatively, many of the activities, including the long-term project, can be completed with other air quality monitors—or no monitor. For example, students can design research projects that utilize existing air quality data instead of collecting their own, which is highly feasible since a great amount of data from around the planet is publically available. In addition, other low-cost monitors could be used instead of the Pods, ranging from purchasable to DIY; see the AQ-IQ unit for a list of options.
Is an air quality monitor needed for this activity?
Yes and No: Yes, if you want the class to collect its own data and become familiar with using the air monitor equipment (especially in preparation for upcoming student projects in this unit), and No if you want to use the provided sample data to just focus on car vs. truck predictions and data analysis, or the chemical reactions that occur during combustion.
Obtaining an AQ-IQ air quality monitor (Pod) from the University of Colorado Boulder
The air quality monitors—called Pods—are available in AQ-IQ Kits that can be rented from the Natural History Museum at the University of Colorado Boulder.
An AQ-IQ Kit is an easily transportable carry-on suitcase-sized container that holds two portable air quality monitors (= 2 Pods), a small laptop for processing data, a comprehensive user manual, and accessories such as power cords and batteries for portable monitoring. The cost is a $10 per week rental fee that supports museum expenses to store and rent the kits. Generally, if students are completing a long-term project, expect to rent a kit for 3-8 weeks.
To find out about the availability of AQ-IQ Kits and shipping options, or to schedule a rental or to rent the kits, contact the museum’s education office at https://www.colorado.edu/cumuseum/programs/schools-and-groups/outreach-materials (a phone number and email address are provided). After checking out the kits, the museum can connect you to a mechanical engineering lab at the university as a technical support resource for using the air quality monitors, troubleshooting and conducting student air quality research projects (as needed). Sometimes the lab partners with schools and provides university undergraduate students to assist teachers with technical issues and to mentor and assist high school students throughout their projects. See the AQ-IQ Program website to learn more and to contact them: http://cuboulderaqiq.wix.com/education.
Copyright
© 2013 by Regents of the University of ColoradoContributors
Ashley Collier; Katya Hafich; Daniel Knight; Michael Hannigan; Joanna Gordon; Ben Graves; Eric Ambos; Olivia Cecil; Victoria Danner; Erik Hotaling; Eric Lee; Drew Meyers; Hanadi Adel Salamah; Nicholas VanderKolkSupporting Program
AirWaterGas SNR Project Education and Outreach, College of Engineering, University of Colorado BoulderAcknowledgements
This material is based upon work by the AirWaterGas Sustainability Research Network Education and Outreach Project in the College of Engineering at the University of Colorado Boulder, supported by National Science Foundation grant no. CBET 1240584. However, these contents do not necessarily represent the policies of the National Science Foundation, and you should not assume endorsement by the federal government.
The authors also express their appreciation for the support of the University of Colorado’s Office of Outreach and Engagement.
Last modified: August 22, 2020
User Comments & Tips